On Hydrogen
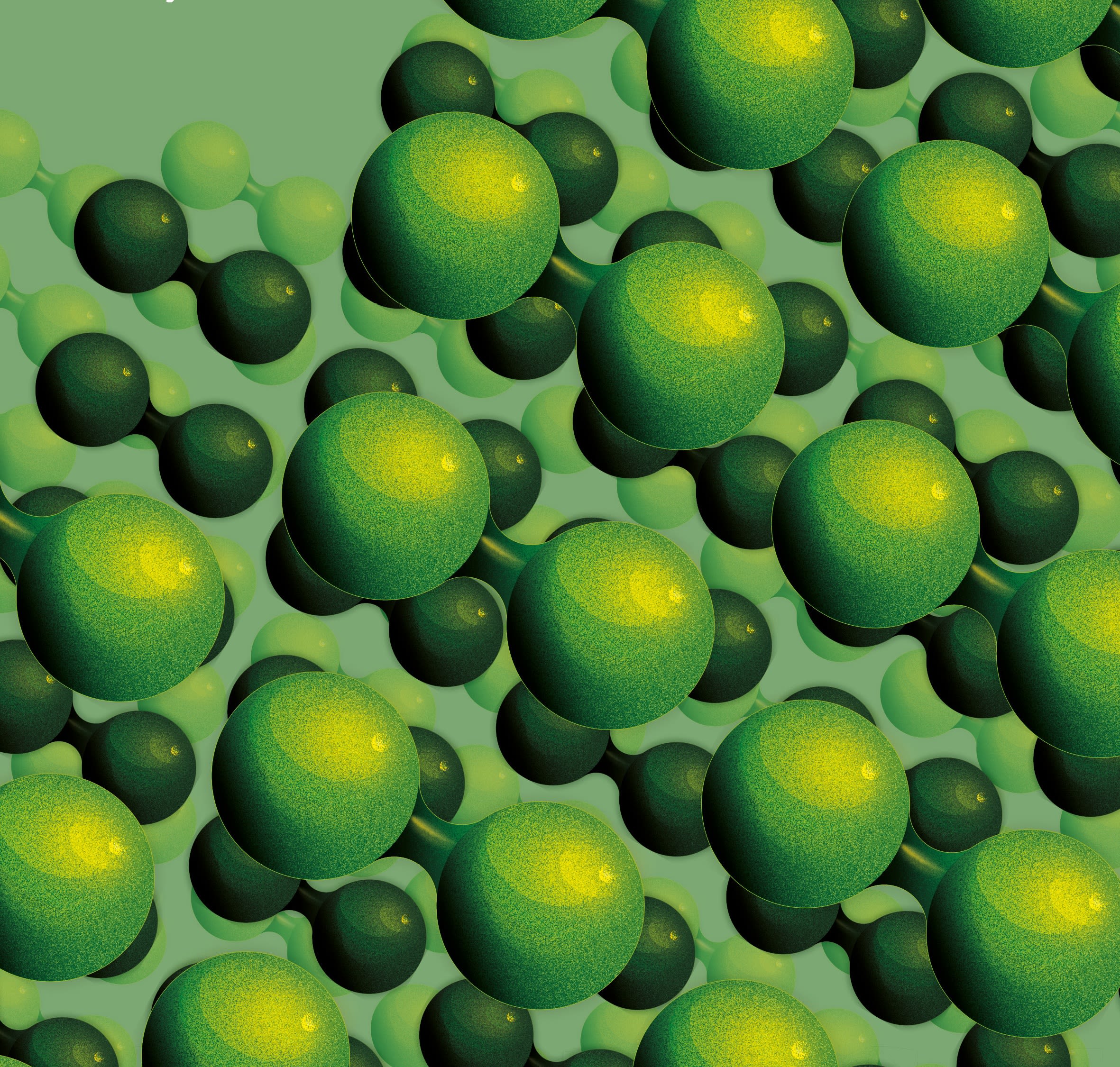
Contents
Foreword
Baroness Brown of Cambridge DBE FREng FRS FMedSci, Chair of the House of Lords Science and Technology Select Committee, Chair of the Carbon Trust, and of the Adaptation Committee of the Climate Change Committee.
How green is hydrogen?
Dr Amanda Lea-Langton
Underground Hydrogen Storage: the key to a green and sustainable future
Dr Lin Ma and Professor Kevin Taylor
Getting it right: are hydrogen standards good enough?
Dr Christopher Jones
Decarbonising transport: is hydrogen the answer?
Professor Alice Larkin and Dr Amanda Lea-Langton
Hydrogen: the missing piece in the clean energy puzzle
Professor Aoife Foley, Dr Dlzar Al Kez and Mr Faraedoon Ahmed
Can hydrogen help decarbonise small industrial emitters?
Dr Vincenzo Spallina and Professor Maria Sharmina
Foreword
The hydrogen bubble has burst. In the last 10 years, hydrogen has been hyped as the silver bullet that can decarbonise almost everything: heating, cars, trains, buses, planes, shipping, industry, electricity generation: all transformed by use of the magic molecule. The trouble with bubbles is that once they have burst, it takes time for industry, governments, and investors to regain confidence and take the subjects seriously again.
And take hydrogen seriously we must. Whilst direct electrification will always be more efficient than using hydrogen produced with green electricity, there will be some key sectors where this green hydrogen will need to be used. The UK Climate Change Committee’s Sixth Carbon Budget’s central scenario for decarbonising the economy has us using 200TWh of low carbon hydrogen in 2050 (from a range of 160-375TWh in other scenarios), equivalent to about 20% of our total energy requirement. When you consider that we use about 300TWh of electricity today in the UK, that means building a low carbon hydrogen industry almost on the scale of our current power system over the next 25 years. And whether it is 5, 10 or 20% of our total energy use in 2050, it is big new industry to build.
The likely uses of low carbon hydrogen are becoming clearer: key industry sectors like decarbonising steel making, producing chemicals including ammonia, making sustainable synthetic fuels for aviation (or indeed fuelling planes with hydrogen itself), shipping and other heavy transport operations, as well specialist applications in mining equipment and excavators. With an electricity grid dominated by weather dependent renewable generation, short and long-term electricity storage become increasingly important, and whilst batteries will cover much of the need for short-term storage, hydrogen stored in salt caverns offers a good solution for storage over periods of weeks, months and even longer.
Plenty of challenges lie ahead in developing a low carbon hydrogen industry. The first is the chicken and egg problem – there are plenty of potential uses and a number of potential routes to producing low carbon hydrogen, but until both potential users and potential producers are convinced that there will be a market for hydrogen at an affordable price, everyone is waiting for someone else to take the first step.
I believe that low carbon hydrogen, especially green hydrogen, can be a green growth opportunity for the UK. We have many of the ingredients to make it work – ambitious net zero commitments and policies; an excellent wind resource and a fast-growing renewables industry, especially for offshore wind; geology such as salt caverns, suitable for storing hydrogen; expertise in electrochemistry in our universities; some serious oil industry players who need an alternative to fossil fuels; innovative fuel cell and electrolyser companies. The National Infrastructure Commission, the Royal Society and the House of Lords Science and Technology Select Committee have all recently stressed the need for strategic long-term energy storage.
The UK Government can take some bold steps to ensure we grow this industry in the UK – it could start by committing to a no regrets investment in a strategic reserve of hydrogen to support our electricity system.
This report provides a range of valuable insights into some of the challenges and opportunities to be gained from a future in which we take low carbon hydrogen seriously.
Baroness Brown of Cambridge DBE FREng FRS FMedSci
Chair of House of the Lords Science and Technology Select Committee, Chair of the Carbon Trust, and of the Adaptation Committee of the Climate Change Committee.
How green is hydrogen?
Dr Amanda Lea-Langton
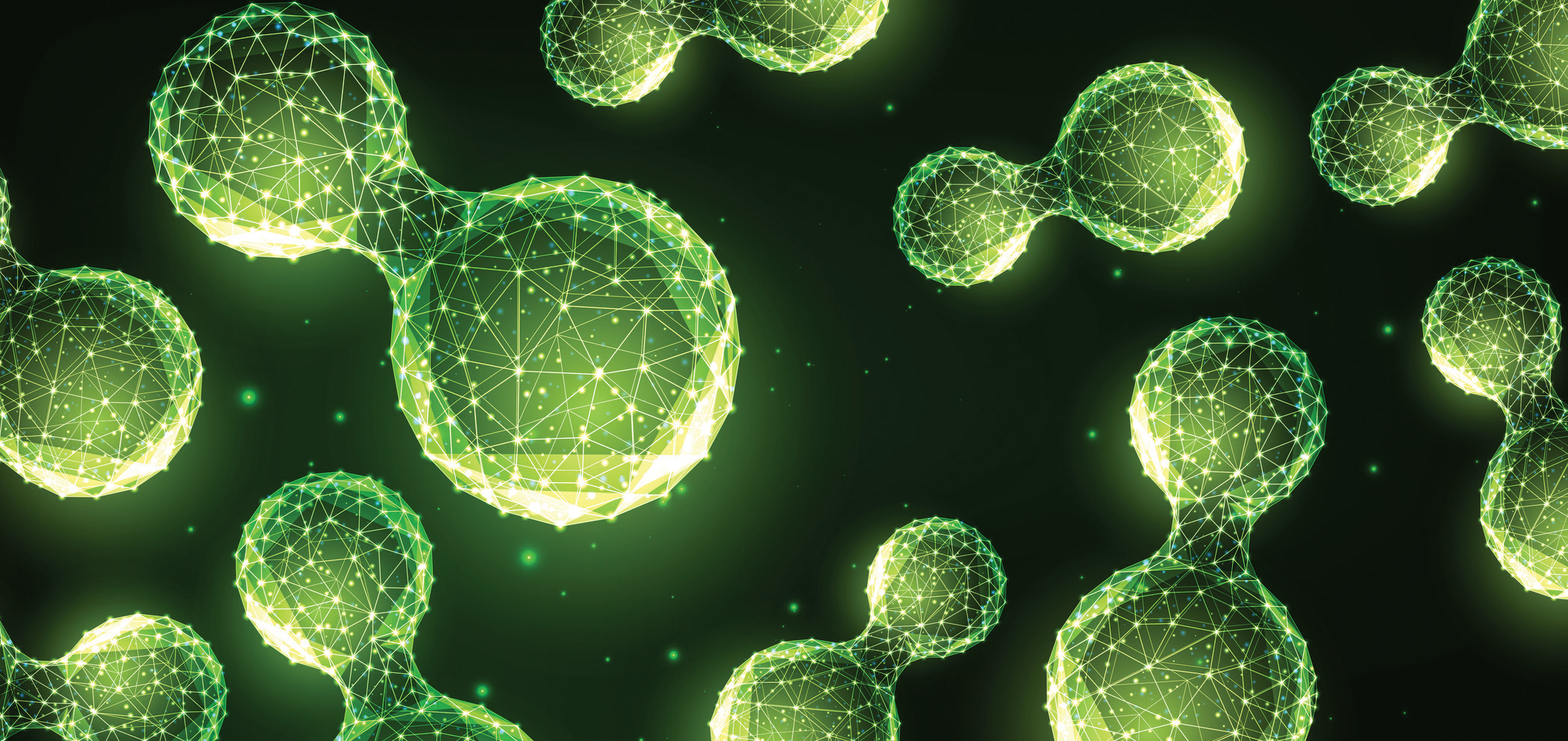
Development of a low carbon hydrogen-based economy is seen as a major part of achieving net zero emissions across the world. Hydrogen is a flexible and potentially non-polluting fuel because it only produces water when it is used in fuel cells or burnt. The energy in hydrogen can be released for use across a vast range of applications from transport to industry and heat.
However, hydrogen is considered an energy storage system rather than an energy source itself. The production of hydrogen can have a high carbon footprint depending on what methods of technology are used. There are many ways that hydrogen can be produced from chemical reactions, electro-chemical methods, and nuclear production.
Using a colour system for identifying hydrogen according to the production process was initially a simple way to distinguish between highly polluting production, for example from natural gas (grey hydrogen) and cleaner, low carbon methods from renewable energy sources (green hydrogen). Over time, the spectrum of colours has become increasingly more complex according to more specific definitions of the production processes, with new definitions and colours, alongside new production options. For instance, green hydrogen is now generally only used for production of hydrogen using electrolysers powered by renewable energy, not for all low carbon technology options. Unfortunately, there is no global agreement on the definitions that are used, so specific production routes are sometimes assigned different colours, leading to confusion.
Colours and production – how hydrogen can be defined
Hydrogen gas is colourless, regardless of how it is made. A typical colour scheme to describe the various feedstocks and production processes used in hydrogen manufacture is shown below, although there are variations used in attribution of these colours.
Black or Brown
Established method of production from either black coal or brown coal (lignite) using gasification. Large amounts of CO2 are released. This is the highest climate impact method.
Grey
Derived from natural gas (methane) using steam methane reforming. This is the most common production type used, with over 90% of hydrogen produced this way. Grey hydrogen has a high climate impact due to CO2 release.
Blue
Typically derived from natural gas (methane) using steam methane reforming, with the addition of carbon capture and storage technologies (CCS) to store the CO2 that is produced. This method still uses fossil fuels and is significantly more expensive than grey hydrogen.
Green
Green hydrogen is split from water using electrolysis, with the energy for the reaction coming from renewable sources such as solar or wind. It is considered to have minimal climate impact as no CO2 is produced from electrolysis.
Purple or pink
This hydrogen is split from water using electrolysis, with the energy for the reaction coming from nuclear power. It produces minimal CO2, but issues of nuclear waste storage and disposal need to be considered.
Turquoise
Produced from the pyrolysis of natural gas or methane. The main by-product is solid carbon rather than gaseous CO2. This method is so far unproven at larger scales.
White
White hydrogen is naturally occurring due to geological processes. Its abundance is unknown but deep geological reservoirs are believed to exist and some mining projects are in operation, however the potential to extract this type without substantial losses is unproven.
The low carbon colour challenge - problems with colour terminology
Hydrogen in its elemental form is extremely rare in the environment, and so energy is required to produce it from other hydrogen-containing materials such as water or methane. More energy is required to produce and store hydrogen than can be recovered by its end use.
The method of manufacturing hydrogen is a fundamental indicator of the hydrogen fuel’s carbon footprint. Using a colour categorisation seems a simple way to understand the climate impacts, however, even within each colour category, the emissions of damaging climate change gases can be very variable.
Most of the hydrogen produced worldwide is in the form of grey hydrogen. This is a high energy and carbon intensive process, so the hydrogen produced in this way cannot be considered a low carbon source. Indeed, research has shown grey hydrogen can have a higher carbon footprint than using the feedstock natural gas directly. There is pressure from conventional energy providers to move towards blue hydrogen, which is the same production process with the addition of carbon capture and storage to mitigate the carbon footprint. Blue hydrogen as an intermediate solution facilitates a continued reliance on fossil fuel feedstock, which comes at the expense of long-term investment in more sustainable alternatives.
Green hydrogen, defined as using electrolysis of water with renewable electricity, offers a genuinely low carbon source. However, sufficient renewable electricity needs to be available at a competitive cost to ramp up its availability. Green hydrogen is extremely expensive compared to the fossil derived options. Electrolysis can also be powered by nuclear electricity production, which is also a low carbon option, although concerns about nuclear waste handling remain a factor.
Some scientists consider mining accumulations of naturally occurring hydrogen (typically ‘white’ hydrogen) as another low carbon source. However, deposits are likely to be deep within geological structures and extraction without substantial losses and environmental damage could be difficult.
It’s got to be green
To catalyse decarbonisation, there needs to be a transition towards low carbon methods over those with high climate impacts A move towards green hydrogen over grey hydrogen will lower emissions, green over blue can lower costs associated with storage. Though there are challenges in terms of production, storage and expense, a straightforward first step would be a set classification of colour terminology which is informed by data on emissions intensity.
Bringing together evidence from research, energy stakeholders and government – including findings from recent hydrogen trials - can ensure colour terminology accounts for specific techniques, the relevant carbon footprints from production, and the threshold of emissions to define each colour, irrespective of the technological methods to simplify hydrogen classification.
In the UK, the Department for Energy Security and Net Zero’s Low Carbon Hydrogen Standard (LCHS) demonstrates an important tool in moving towards consensus on low carbon hydrogen. A further refresh of this standard, to include agreement around the intensity of emissions that demarks a particular colour classification or a move solely to an emissions intensity classification, is crucial to ensure low carbon delivery. However, to deliver the most impact, these classifications will need to be harmonised not only nationally but internationally too. The previous government acknowledged that a next step to introducing the LCHS would be to work on a pathway to international alignment for standards and certification. This might include working with stakeholders like the International Energy Agency and the International Organization for Standardization. The Department for Energy Security and Net Zero should convene an international working group to progress this important pathway to alignment and demonstrate leadership.
The previous government also made a start on moving away from high carbon impact hydrogen with the announcement of the largest number of commercial scale green hydrogen production projects. Policymakers must now build on this, ramping up investment and incentives for renewable electricity to support the production of low carbon green hydrogen over fossil fuel driven and CCUS-enabled blue hydrogen. Scaling up the UK’s hydrogen economy can only be achieved if green hydrogen is affordable and abundantly available.
About the author
Amanda Lea-Langton is a Senior Lecturer in Bioenergy Engineering and lead for Environmental Energy at the Manchester Environmental Research Institute (MERI), The University of Manchester.
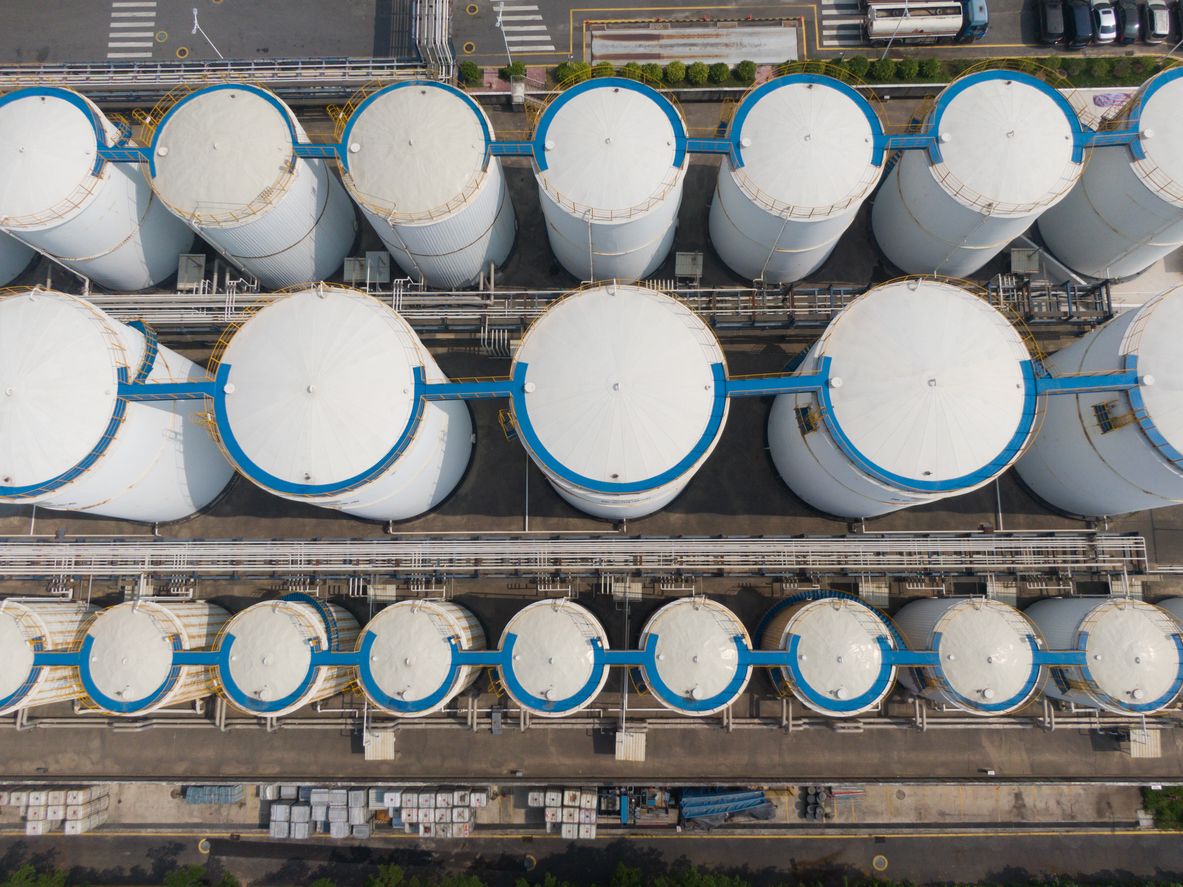
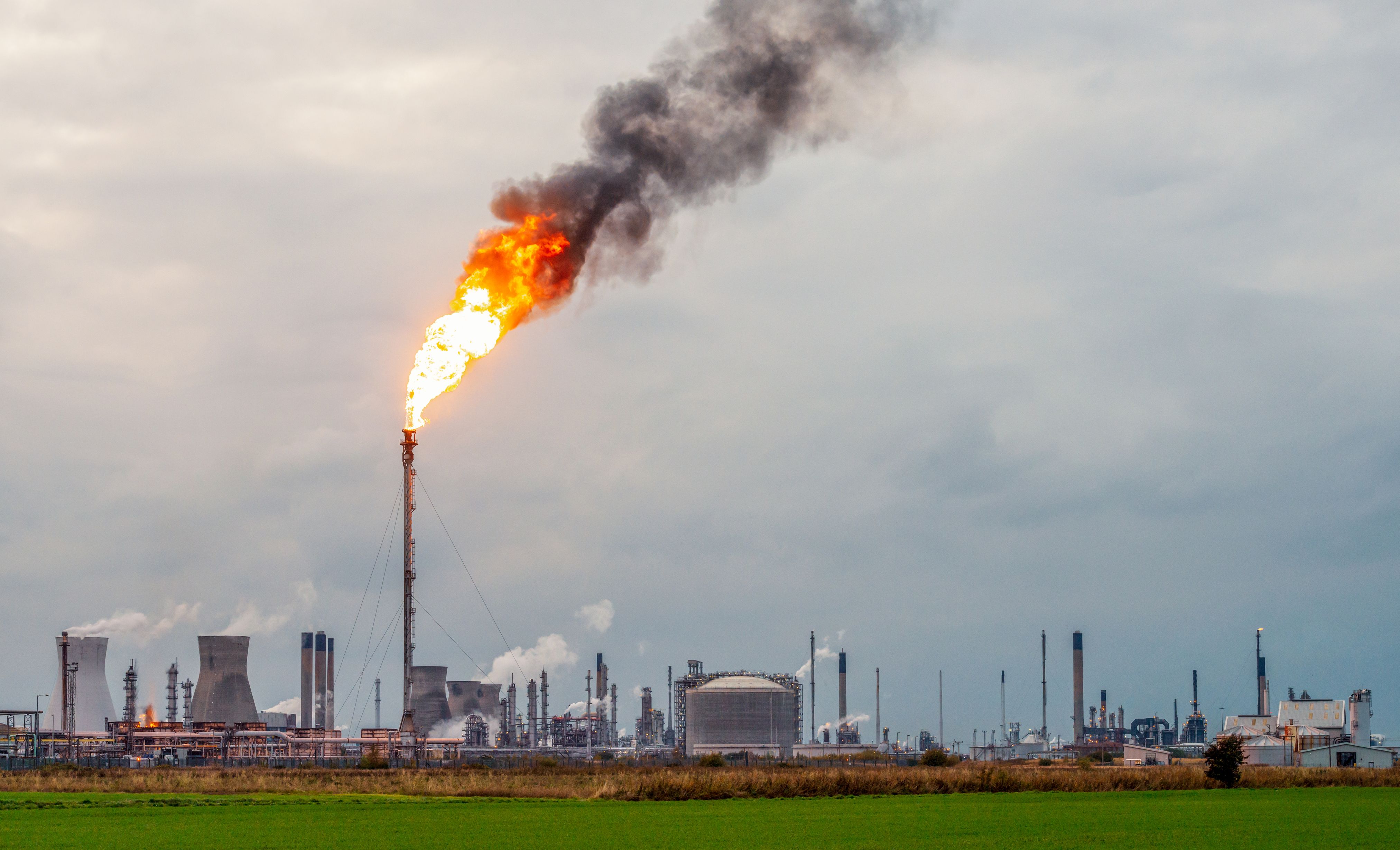
Is advanced nuclear the route to low-carbon hydrogen production?
Dr Will Bodel
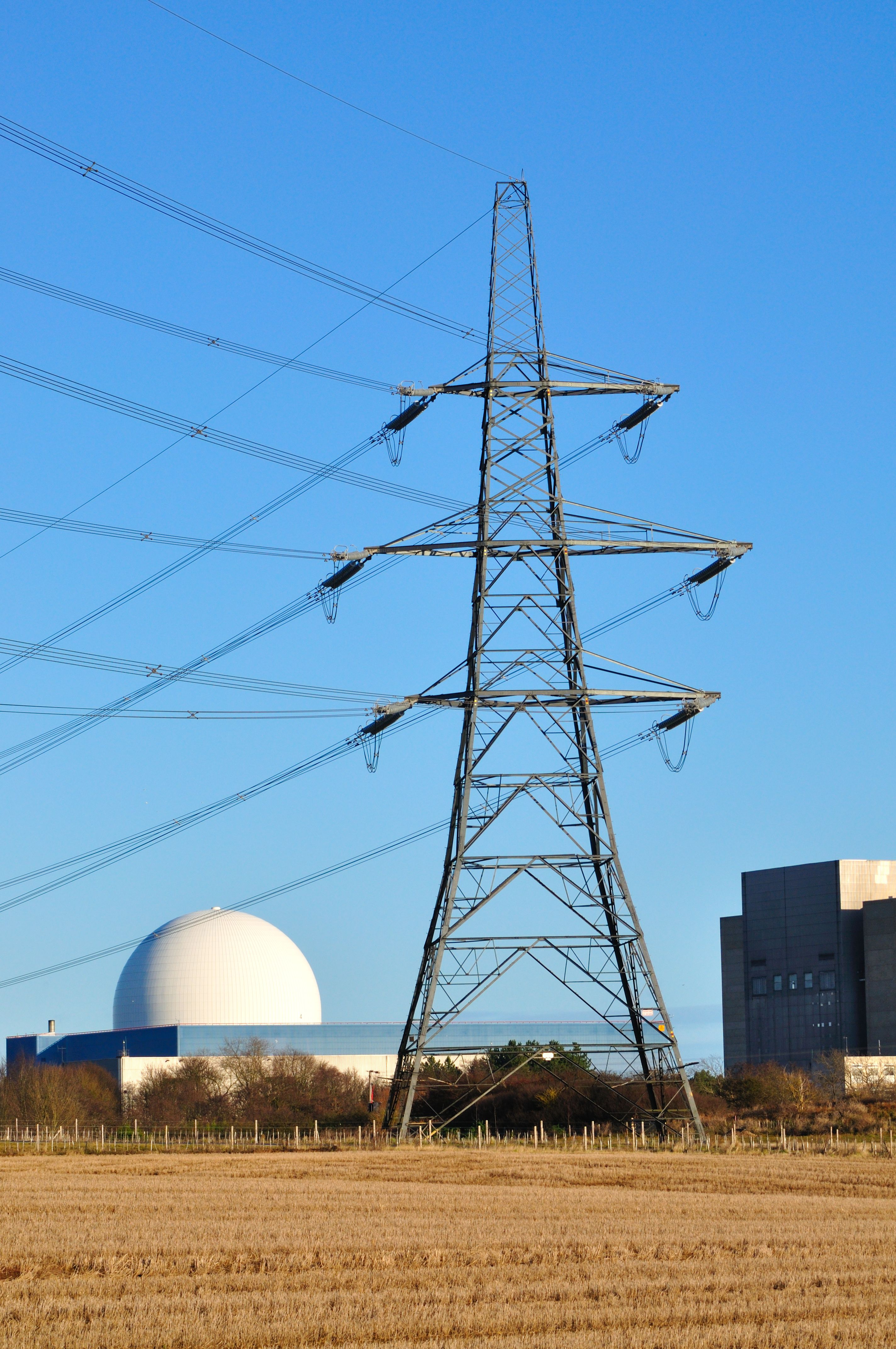
There is increasing recognition that a UK net zero future will feature a significant role for hydrogen as an energy vector. The UK currently relies heavily on natural gas for heat, industry and power production. If net zero ambitions are to be realised, this must be replaced with either increased electrification (powered by low-carbon sources) or an alternative low-carbon fuel; hydrogen seems to be the leading contender for the latter. But large-scale, clean, hydrogen generation requires a vast amount of low-carbon energy right across the production life cycle, if it is to be helpful for carbon reduction.
Many large-scale hydrogen production routes are under consideration, with enough ‘colours’ assigned to them to resemble a paint colour chart, each of them requiring a huge amount of energy. At the Dalton Nuclear Institute, we believe that next-generation advanced nuclear reactors offer a uniquely effective means to do this. Here, we consider two reasons why.
Making nuclear flexible
Firstly, we must consider what an effective net zero electricity grid would look like. The grid will be larger than today’s and made up of low-carbon sources. The only scalable low-carbon options at present are renewables (in the form of wind and solar) and nuclear. In the previous government’s plans for the future, renewables make up the bulk of electricity generation, but these have the drawback of being variable. For the periods where the sun doesn’t shine much and the wind doesn’t blow much, a flexible alternative technology is needed to fill in these gaps and meet the nation’s power demand.
Today’s nuclear plants don’t fit that role very well. While cheap to run, they are expensive to build, which makes the concept of a nuclear power station sitting idle for extended periods, when its power isn’t needed, extremely bad economically. The economics of nuclear plants are therefore optimised by running them at full power, 24/7. In contrast, most of the cost for natural gas power generation is the cost of the fuel. Gas plants are also quick and easy to power up and down, making them ideal for filling in gaps in generation elsewhere.
“Nuclear is inflexible, so delivers baseload power” has long been an established truth for electricity generation but introducing hydrogen production - as well as electricity - to the mix, creates a way to make the output flexible, diverting nuclear output towards electricity or hydrogen production as required. This means reactors can run at full output capacity, and their energy split between the grid or hydrogen production according to demand. Even rapid changes in renewable output can be accommodated by turning the hydrogen production ‘dial’ up and down to divert more or less nuclear energy to that purpose.
Efficiency
The second reason nuclear power is emerging as a preferred route to hydrogen production relates to the way hydrogen is produced. Although hydrogen is abundant on Earth, it doesn’t exist in any significant quantities in pure elemental form, so it needs to be converted from something which contains hydrogen – usually methane (CH4) or water (H2O). Conversion requires a lot of energy in the form of heat and/or electricity.
The vast majority of the world’s hydrogen is currently produced from steam reforming natural gas. This has the downside of producing carbon dioxide in addition to hydrogen – counter-productive when the motivation for using hydrogen is to reduce emissions. Economics are also dependent on gas prices, which in the future may be higher than in the past.
Electrolysis (essentially water + electricity → hydrogen) provides a potential alternative, so long as the source of the electricity is both affordable and low carbon. Electrolysis can be broadly split into low - and high - temperature processes, with the latter particularly relevant for nuclear. This utilises Solid Oxide Electrolyser Cell (SOEC) technology, which is developing rapidly, with operating temperatures expected to reduce to below 650°C. This puts the process well in range of the operating temperature of many advanced reactor designs, including the high temperature gas cooled reactors (HTGR), which the previous UK government committed to demonstrating by the 2030s. For comparison, current light water reactors operate at around 300°C.
High temperature electrolysis is likely to be the cheapest route to hydrogen production, provided the high temperatures it requires are available. This makes it an ideal technology to partner advanced reactors. Such reactors can provide a variable mixture of high-temperature heat and electricity to a hydrogen production facility, in addition to putting electricity into the grid when needed. By delivering vast quantities of high-temperature heat direct to the process, both the additional demand for electricity and the overall cost of the hydrogen production are significantly reduced.
A feasibility report for Department for Business, Energy and Industrial Strategy (BEIS) found overall process efficiencies of over 50% can be achieved for the generation of hydrogen using advanced reactors, compared to efficiencies of less than 40% for low temperature electrolysis. This improvement in overall economics could have a massive impact on the viability of bulk, low-carbon hydrogen production.
Our recent research at the Dalton Nuclear Institute demonstrates how renewables and nuclear working in tandem – alongside a route to hydrogen production – can both reduce emissions by eliminating the use of backup gas-fired generation and save money by reducing the costs of backup plant or expensive energy storage, allowing both nuclear and renewables to operate to their full potential.
Policy recommendations
Reaching net zero will require huge amounts of low-carbon electricity, much of it from renewable sources. Without an effective means of balancing their variable output, we will need a vast over-supply of renewables, which will mean curtailing that output much of the time (turning turbines or solar panels off when demand is lower than the available supply). Having advanced nuclear as a balancing technology not only allows us to generate substantial amounts of hydrogen at a competitive cost, but it also allows renewable technologies to operate to their full potential, without curtailment.
High temperature advanced reactors are particularly suited to generating hydrogen and should be built at scale. These should be equipped for delivering hydrogen and electricity generation for the grid. This would enable them to generate electricity for the grid when output from other low-carbon generators is low. We recommend that government continues to support the delivery of advanced nuclear – at accelerated pace and with full recognition of the wider benefits to achievement of net zero.
Currently, government modelling of low-carbon futures takes a siloed approach – considering heat, hydrogen, renewables and nuclear independently. We recommend a much more integrated view be taken in forward planning, recognising the important interconnections and potential benefits which can result. This will allow renewables and nuclear to work together for a win/win outcome.
About the author
Will Bodel is a Fellow in Nuclear Energy Policy at The Dalton Nuclear Institute, The University of Manchester.
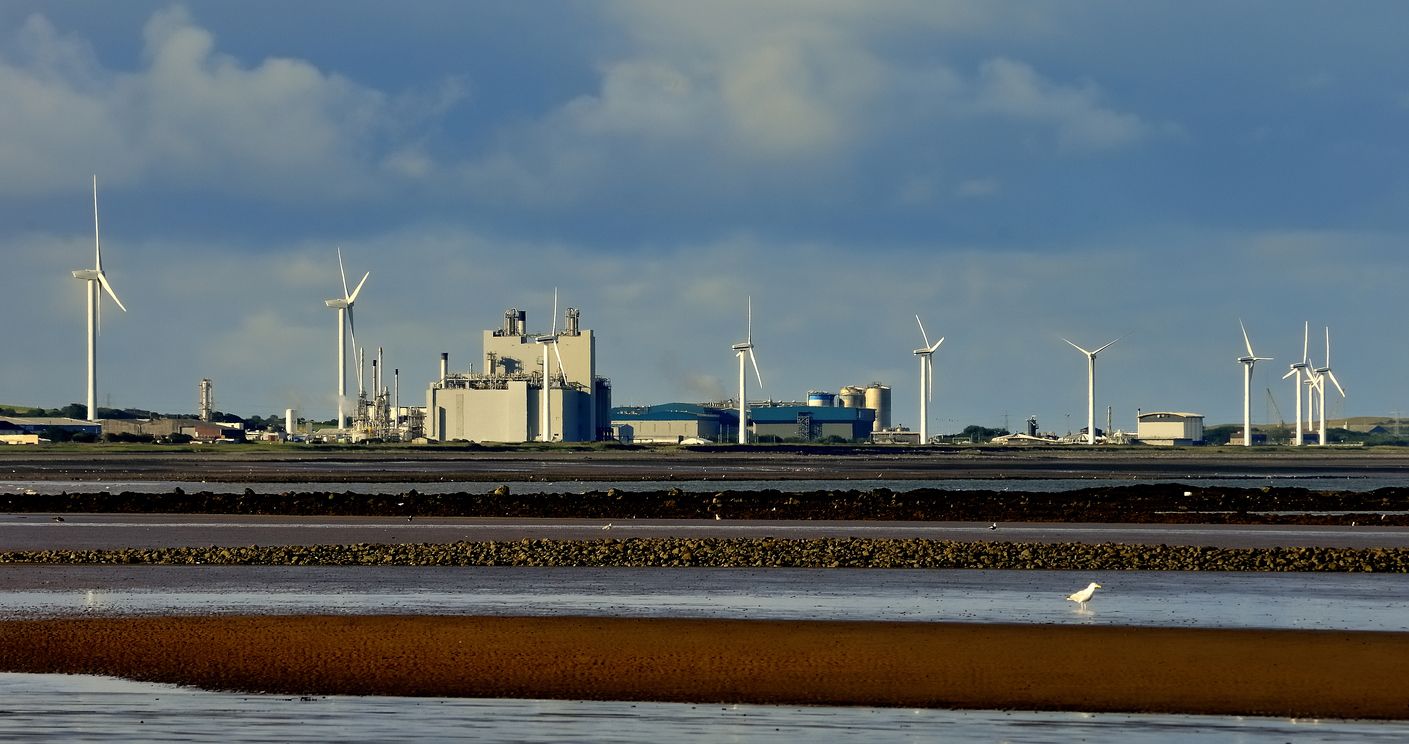
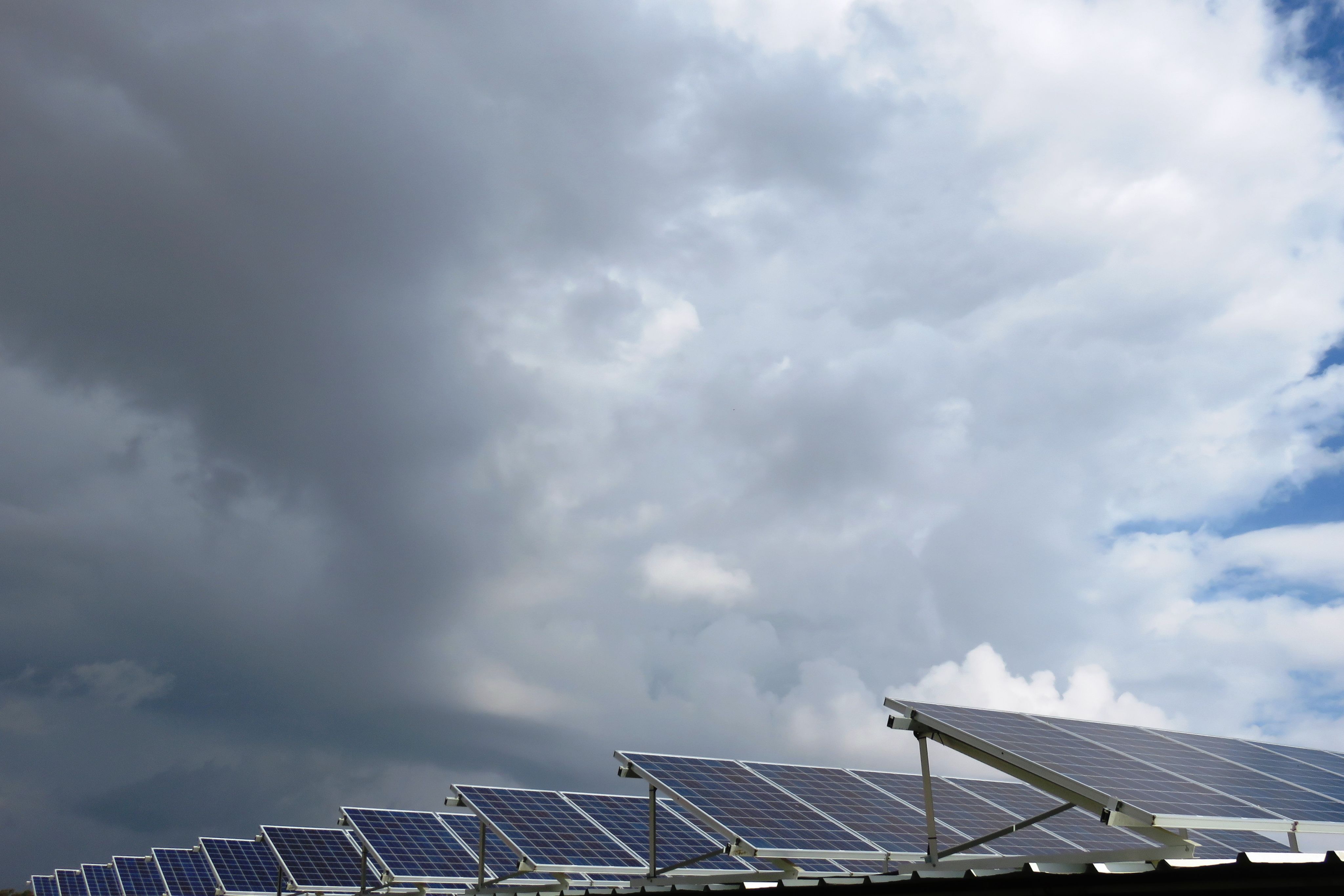
Underground hydrogen storage
the key to a green and sustainable future
Dr Lin Ma and Professor Kevin Taylor
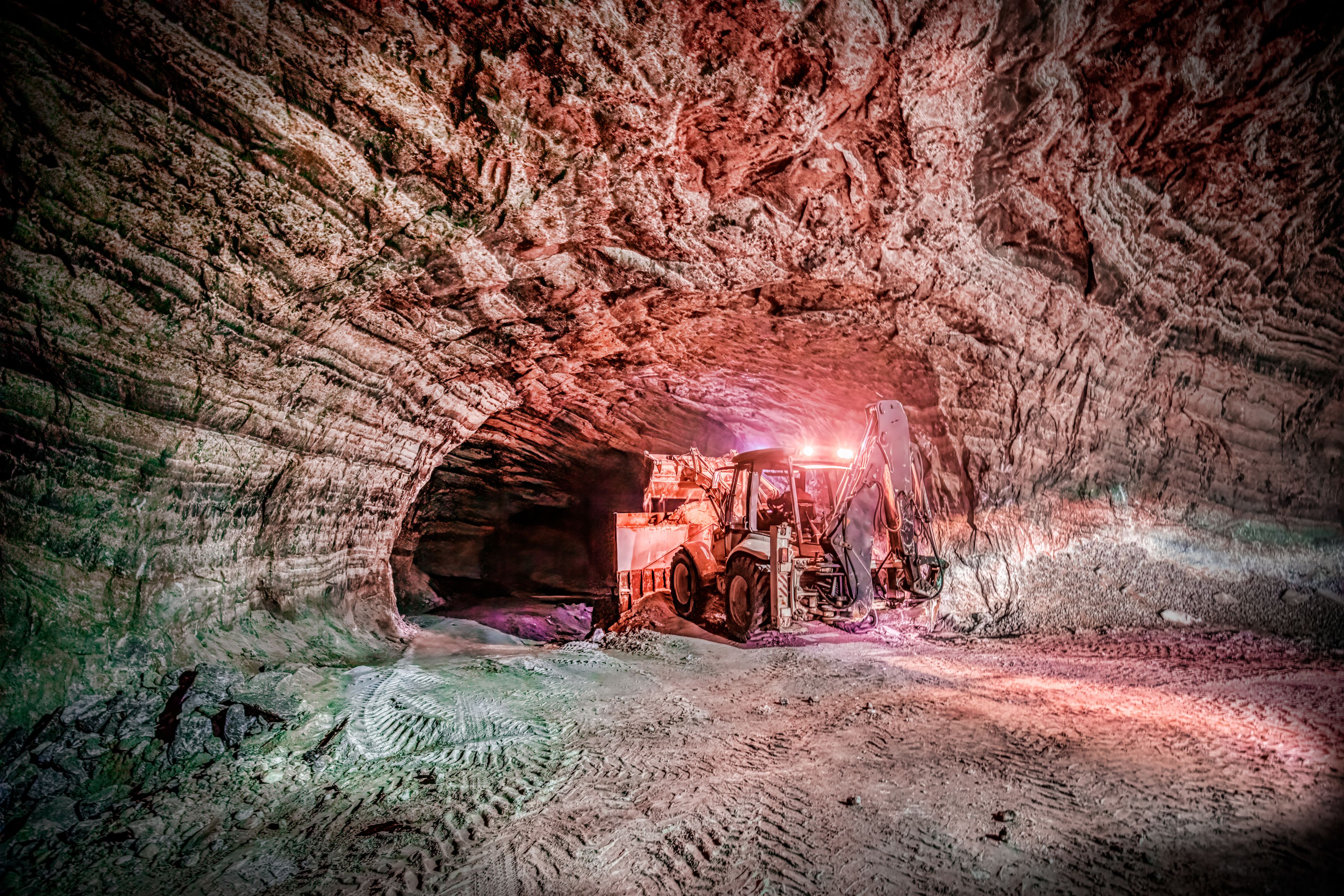
In response to the climate crisis, renewable energy production has increased significantly over the last decade. According to National Grid, the UK produced its trillionth kilowatt hour (kWh) of electricity generated from renewable sources on 15 May 2023, and solar energy and offshore wind are predicted to grow five-fold by 2030. Hydrogen, a potentially green energy carrier, is also seen to be increasingly important as part of UK energy decarbonisation.
Hydrogen is one of a handful of new low carbon solutions which can help the UK to achieve its emissions reductions target for Carbon Budget Six (CB6), and net zero by 2050. However, if hydrogen is to significantly contribute to our energy mix, then its storage will form an important part of the supply chain, and there are a number of associated challenges that policymakers will need to address.
Hydrogen and Underground Hydrogen Storage (UHS)
The previous UK government’s Ten Point Plan for a Green Industrial Revolution aimed for 5GW of low carbon hydrogen production capacity by 2030, for use across the economy. Hydrogen could be a key element in helping to achieve net zero as it has the potential to be used to decarbonise heavy industry, transport, and heating. Hydrogen storage is an important part of the supply chain, allowing excess renewable energy to be stored in various formats.
In the UK, 3.4 TWh of large-scale hydrogen storage is predicted to be required by 2030, increasing to 10 TWh by 2035. Large-scale hydrogen storage will be the only realistic way to balance seasonal fluctuations of renewable energy and customers’ demands. UHS has been proposed as the most promising large-scale storage approach to provide ~10GWh scale storage capacity. Here, electricity produced by renewable energy will be transformed to hydrogen by electrolysis (so-called green hydrogen). Gigatons of hydrogen could be generated at peak times of renewable energy production, in the summer for instance, and stored until it is needed in peak times of energy demand, like in winter. The stored hydrogen will be extracted from the subsurface as hydrogen gas and utilised.
Methods of UHS
Hydrogen can be injected and stored in a variety of geological structures including salt caverns, depleted gas reservoirs, or depleted aquifers (materials such as porous rocks that store water underground).
The safety and efficiency of hydrogen storage in salt caverns has been proven in several locations globally. Examples include the Teesside salt field, providing 25GWh capacity since 1972, and three salt domes - Clemens Dome, Moss Bluff, and Spindletop, in Texas - providing 90 to 120 GWh capacity since the 1980s. However, this type of storage will struggle to meet the rapidly increasing need for hydrogen due to their restricted geological distribution.
Depleted gas reservoirs could provide high volumes of UHS due to the porous nature of the rock and have proven ability to store and trap natural gas for millions of years. However, to date, pure hydrogen has never been stored in depleted gas reservoirs.
Deep aquifers could potentially store even higher amounts of hydrogen owing to their wide distribution and large volumes. However, deep aquifers require further assessment to understand whether their sealing ability is sufficient for hydrogen.
Challenges faced by UHS
Before UHS can play a full role in the supply of hydrogen, there are still many scientific challenges that need to be addressed.
The small molecular size of hydrogen makes it moveable and migratable through porous rocks or fractures, potentially leading to the escape of hydrogen into the subsurface. A careful assessment of effective seals and containment mechanisms is critical to prevent leakage and maintain the integrity of the storage site.
While storing and recovering hydrogen from the subsurface, some energy is required for compression, injection, and extraction processes. Although challenging, improving the efficiency of these processes is important to maximise the energy storage potential and overall viability of UHS systems.
The interaction between hydrogen and the subsurface environment can lead to geochemical and microbiological reactions. These reactions may affect the integrity of the storage reservoir, alter the chemical composition of the surrounding rocks or fluids, and potentially impact the long-term storage capacity. Managing these reactions are crucial for maintaining the stability and safety of underground hydrogen storage.
UHS involves injecting and withdrawing large volumes of hydrogen, which can induce mechanical stresses on the storage reservoir and surrounding rocks. The mechanical response of the reservoir to these stresses, including the potential for induced seismicity or subsidence, needs to be carefully evaluated and monitored to ensure the safe operation of the storage facility.
Environmental impacts of UHS must also be assessed and mitigated. This includes evaluating potential risks associated with hydrogen leakage, such as its impact on groundwater quality, soil contamination, or air quality. Robust monitoring and mitigation strategies are essential to prevent and respond to any environmental concerns that may arise.
The deployment of UHS facilities will have social implications that need to be considered, such as concerns related to land use, community acceptance, and public perception of underground storage technologies. Engaging with local communities, addressing concerns, and ensuring transparency in the decision-making process are all important for fostering acceptance and support for UHS projects.
Extensive research, pilot projects, and thorough risk assessments are necessary to develop safe, reliable, and environmentally sound UHS solutions. Scientists at The University of Manchester are driving the development of novel technologies and are delivering cutting-edge knowledge to the new areas of UHS, together with low-carbon hydrogen production techniques, to ensure a sustainable future.
Policy recommendations
A holistic approach is required to be taken by government and other policymakers to ensure our hydrogen networks are able to supply the energy we need by 2035.
Initially, research and development must be supported by further investment to help accelerate the progress of energy transition. Research and development of UHS is at a relatively early stage and the scientific challenges outlined above need to be addressed before it can become fully operational. These challenges will only be addressed through multi-disciplinary research undertaken by academics and industry.
Policies that encourage and regulate industrial development should be introduced. This includes setting targets for renewable hydrogen production, implementing carbon pricing mechanisms, providing financial incentives and subsidies, and removing barriers to market entry. This will help to stimulate market demand and encourage the private sector business to step into the market.
The government should also collaborate with other nations to promote development of a global low-carbon hydrogen market involving UHS. This cooperation could involve sharing best practices, harmonising standards, and regulations, establishing cross-border infrastructure for hydrogen production, storage, transportation and trade, as well as resource sharing and knowledge exchange, which could accelerate progress in scaling up hydrogen storage technologies.
Finally, public acceptance and understanding of UHS is also vital for a successful transition to a hydrogen-based economy. The government and industry can work together to increase public awareness through educational campaigns, public demonstrations, and providing accurate information on the environmental and economic advantages of low-carbon hydrogen.
About the authors
Lin Ma is a Senior Lecturer in Chemical Engineering in the School of Engineering at The University of Manchester.
Kevin Taylor is a Professor of Geoscience in the Department of Earth and Environmental Sciences at The University of Manchester.
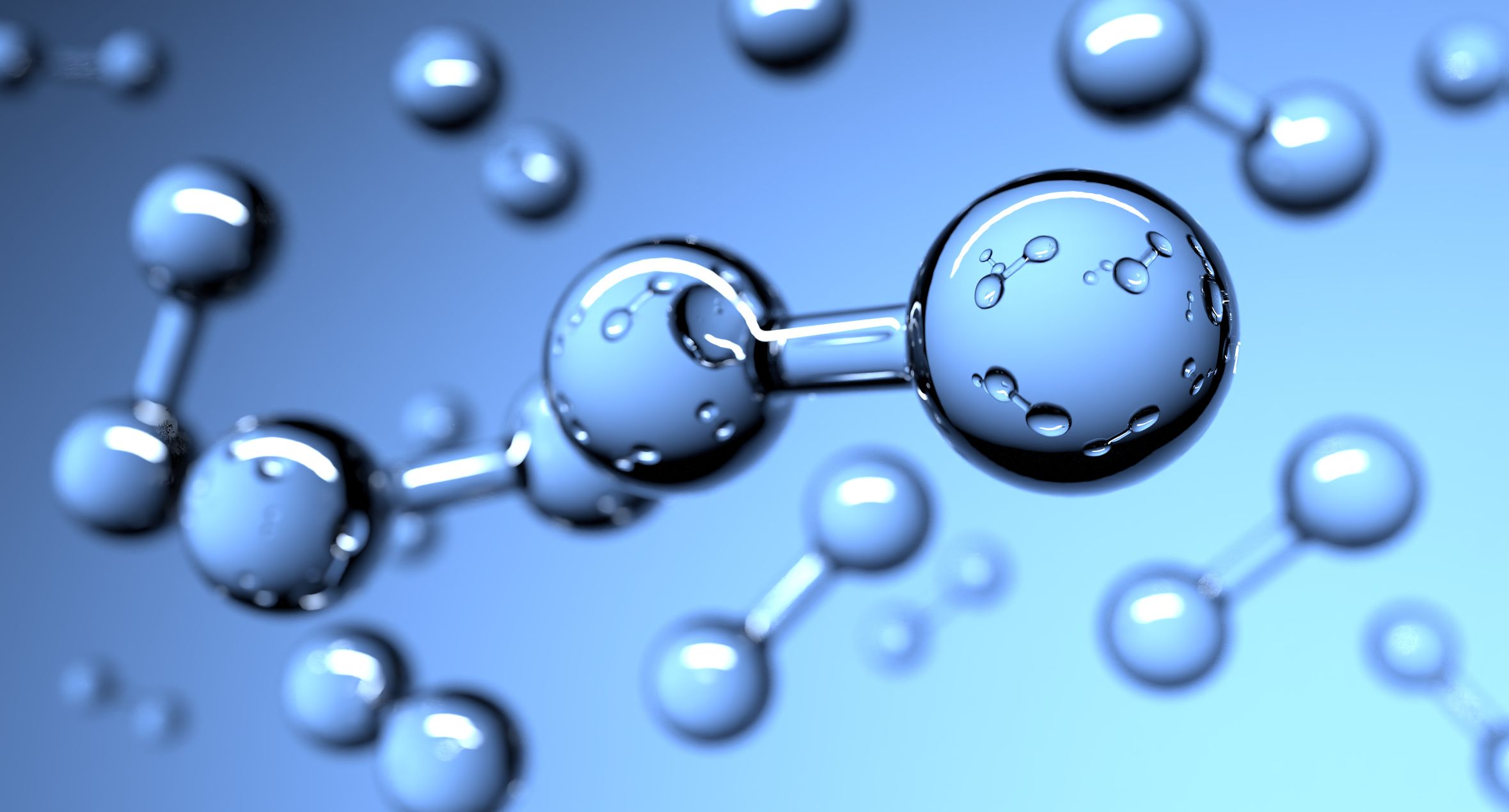
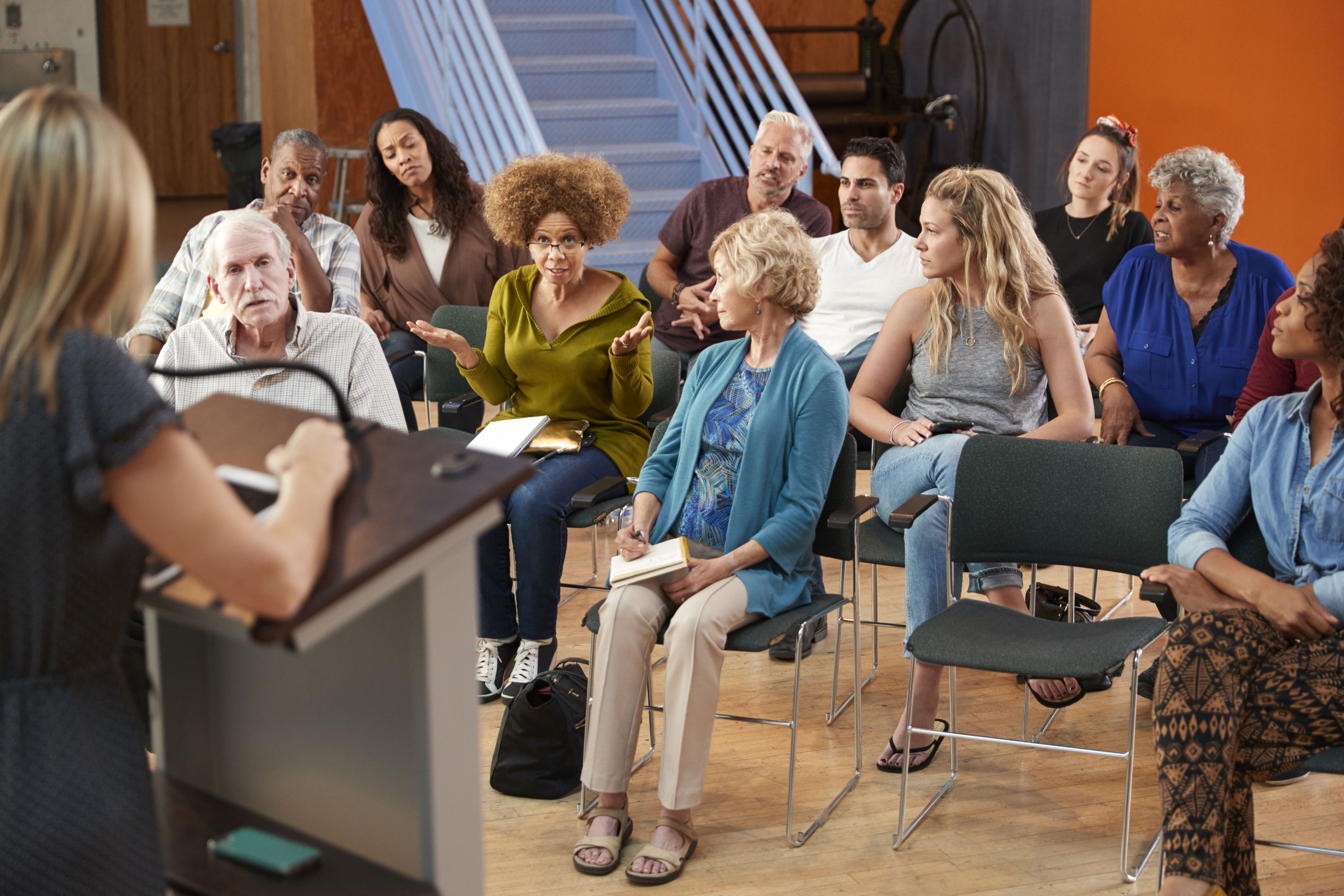
Getting it right
are hydrogen standards good enough?
Dr Christopher Jones
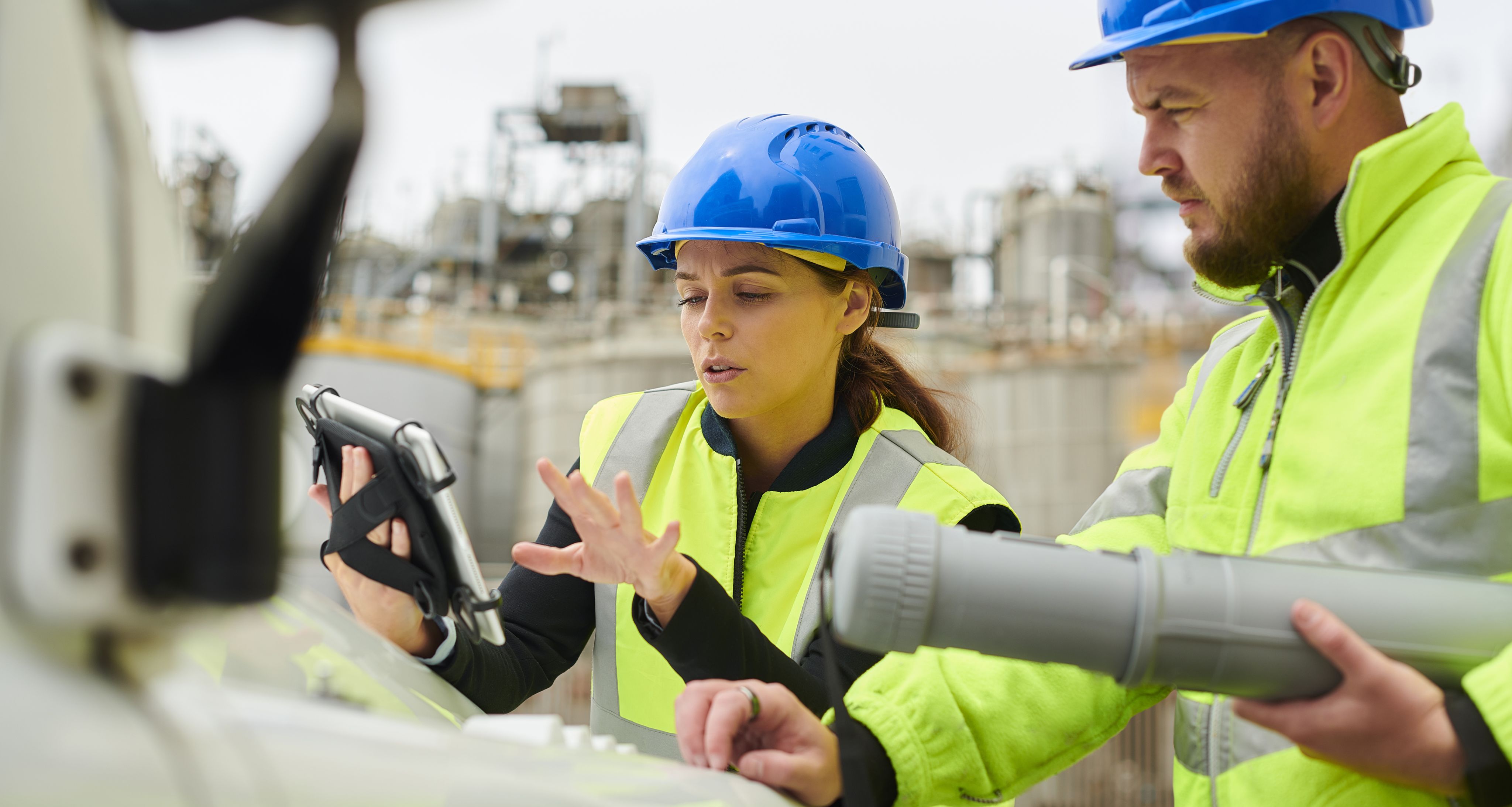
Hydrogen has the potential to be an important industrial feedstock and fuel. There are, however, various ways to produce and use it, leading to diverse supply chains with different environmental implications. Two considerations for the role of hydrogen in a low carbon economy stand out - ensuring that hydrogen production and supply has sufficiently low impact on the climate and using hydrogen in an optimal way.
Hydrogen production
Hydrogen has multiple production means. It can be produced via electrolysis or stripping hydrogen from natural gas or a biomass feedstock, but there are several variations within these processes.
Thermal treatments of natural gas (grey hydrogen) account for 99% of current production and have high carbon emissions.
Capturing and storing the carbon released through this process (blue hydrogen) should lower most of these emissions but with elevated ‘fugitive’ greenhouse gas (GHG) emissions from sourcing more natural gas.
Electrolysis separates hydrogen from water, so no carbon is realised by the reaction – its environmental impact is largely determined by the source of electricity, whether renewables (green hydrogen) or the grid average. Gasification processes with biomass inputs are also in development for hydrogen supply.
Low carbon hydrogen standards
The UK, US, EU and others have set low carbon hydrogen standards. In the UK’s case, this means for government funded projects where GHG emissions are measured ‘well-to-gate’, (i.e. across the production process but not including hydrogen transportation and storage), emissions should not exceed 2.4 kgCO2e/kg of hydrogen.
This is particularly important for blue hydrogen, where there is significant uncertainty about the overall GHG impact for production. It takes more natural gas to provide a Megajoule (MJ) of blue hydrogen than a MJ of natural gas used directly. Emissions from natural gas extraction and processing have an important climate impact that the industry has so far not managed to curtail.
How far blue is from grey hydrogen rests largely on how much CO2 is captured in production. The UK Environment Agency guidance requires capture rates of 95% or more, but there is little data on actual capture rates for blue hydrogen, with projects not yet meeting these requirements consistently.
These standards are important for ensuring future hydrogen does what we need it to, but currently they only apply to government supported schemes and minimum capture rates are guidance as opposed to law. Current UK standards also do not encompass downstream emissions from the transport and storage of hydrogen or the role of hydrogen as an indirect GHG.
Comparing standards internationally
The EU definition for low carbon hydrogen (3.4 kgCO2e/kg) includes downstream emissions. For context, one study for the UK government puts potential downstream emissions (for the period up to 2030) at 0.7 to 2.3kg. While there are monitoring and evaluation benefits of limiting to well-to-gate, it does mean that the UK standard can allow for more GHG emissions over the life cycle of hydrogen and does not sufficiently prioritise the lowest carbon option. The US standard sets a maximum well-to-gate emissions value of 4 kgCO2e/kg of hydrogen, but also offers progressively larger tax credits from 3 to <1kgCO2e/kg.
None of these current standards account for hydrogen as an indirect GHG when leaking into the environment, and its effect on the abundances of methane, ozone, stratospheric water vapour and aerosols. Quantifying the impact of leaked hydrogen emissions is challenging but the effect may be significant, therefore it is crucial to minimise leakage.
More data is needed to fully quantify leakage potential, particularly over large distribution networks, as proposed for hydrogen home heating. Improvements in sensor equipment and more studies on losses in different pipe configuration, storage and handling processes would allow for more informed judgement of hydrogen’s full climate change impact potential.
Prioritisation
Around 95 Mt of hydrogen is produced annually and is predominantly used for making fertiliser and petrochemicals. Future demand is expected to grow for these traditional uses and for new energy sector applications, like industrial processes and transport. The gap between production capability and foreseen demand makes it important to consider where hydrogen is best used. There are several ways to do this, for example through the use of the Hydrogen Ladder. But there must also be consideration of alternatives and the suitability of hydrogen for the role. Decision makers must therefore prioritise where new hydrogen production will have the greatest impact on sustainability - for instance, replacing high carbon hydrogen industry feedstocks or home heating, where more effective alternatives already exist for the latter.
If blue hydrogen cannot meet environmental criteria on production emissions when standards are enforced, then green hydrogen will be the predominate form of production. In this case, production may follow where renewable electricity is available at lowest cost. This may introduce new international trade between countries looking to become green hydrogen exporters (e.g. Australia, Chile and Morocco) and regions with growing markets for hydrogen such as Europe and Japan.
Stronger regulations and better standards
Strong and aligned regulation that considers hydrogen transport, storage emissions, as well as hydrogen leakage over the supply chain is needed for environmental safety, but also to provide investor confidence. The majority of planned low carbon hydrogen production capacity is stuck at the final investor decision stage awaiting clearer policies on regulations and incentives from future consumers like the UK. Policymakers can ensure that the UK is well placed to attract investment and provide such confidence by having comprehensive regulations that are reviewed regularly to incentivise only the lowest carbon options for hydrogen. This would take into account technological advances, new research and evidence, and allow for stakeholder feedback.
Governments are already taking positive steps to ensure new hydrogen production is suitable for the low carbon future, but these standards should be tighter, mandatory for all new hydrogen producers, and should account for hydrogen leakage.
Tightening standards would mean expanding the scope of UK, US and Japanese definitions for low carbon hydrogen to include transportation and storage, and for the UK, EU and Japan, enhancing incentives for very low GHG emission H2 as proposed in the US. Mandatory standards set by the Department for Energy Security and Net Zero could be applied in a similar way to the Renewable Transport Fuel Obligation, as is the approach for renewable hydrogen in the EU. Tighter standards would also include a commitment to monitor hydrogen leakage and then develop a robust standard which minimises it.
The issues around whether sources of hydrogen are low carbon enough, and available to meet demand beyond existing crucial uses such as fertiliser, have implications for policymakers planning for a low carbon transition. While markets may adjust themselves to prioritising the allocation of low carbon hydrogen to sectors with fewer alternatives, policymakers should take the approach of routinely reviewing the hydrogen sector and plan ahead to ensure priority sectors can source hydrogen safely and effectively. This needs to happen in a timely manner to avoid further delays to investment in low carbon hydrogen and in alternatives to hydrogen where decision makers are unsure whether to electrify or await hydrogen options.
About the author
Christopher Jones is a Senior Knowledge Exchange Fellow at the Tyndall Centre for Climate Change Research, The University of Manchester.
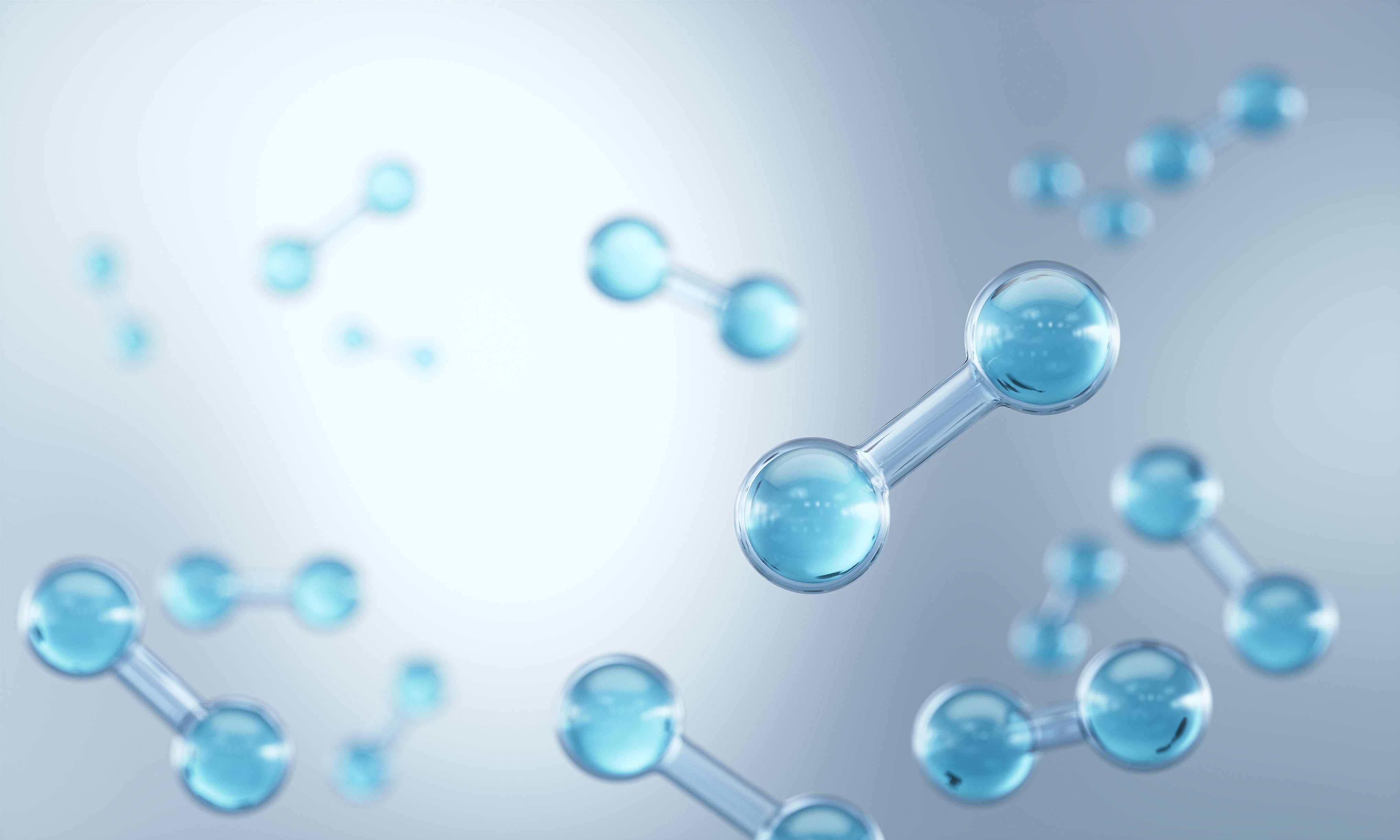
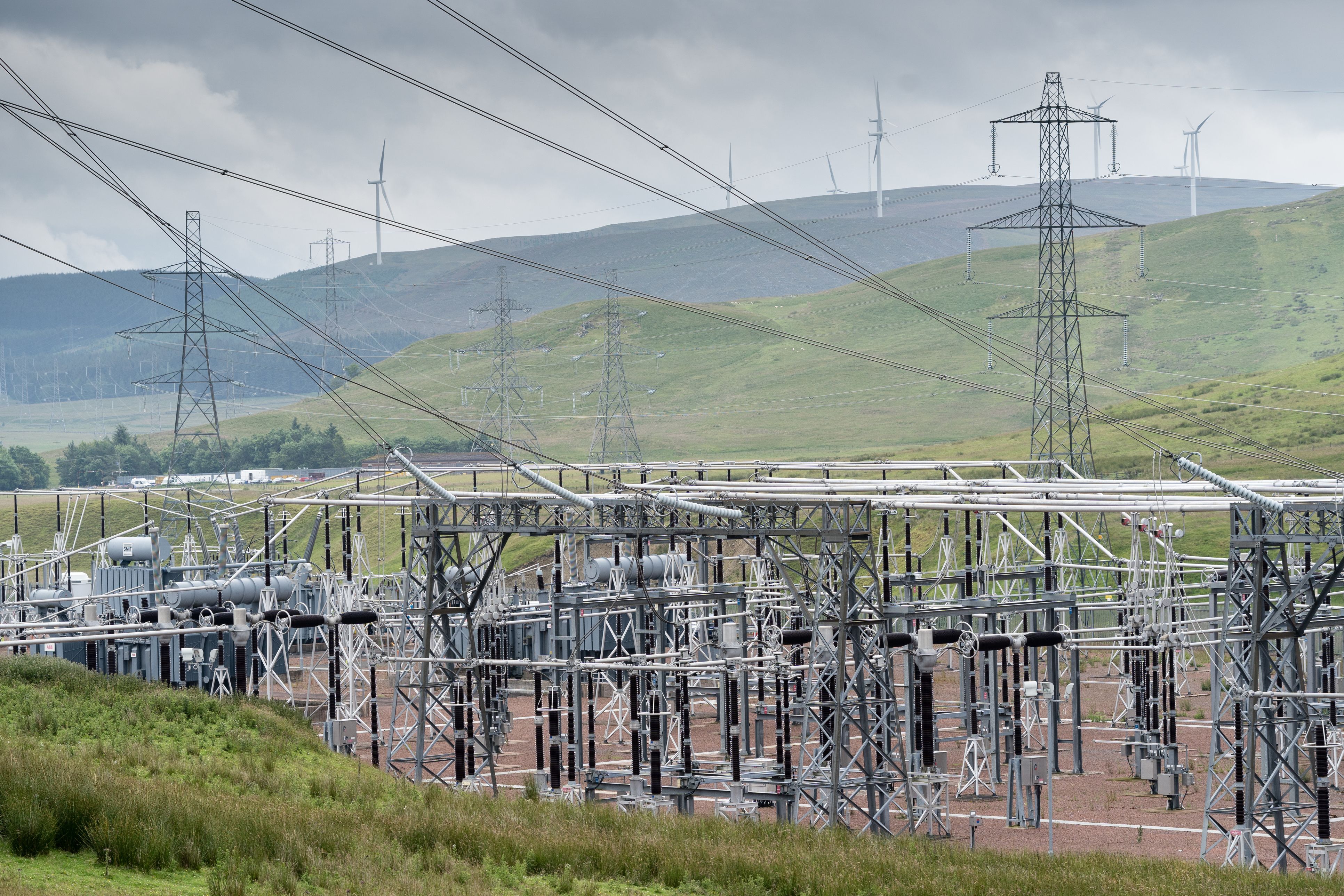
Decarbonising transport
is hydrogen the answer?
Professor Alice Larkin and Dr Amanda Lea-Langton
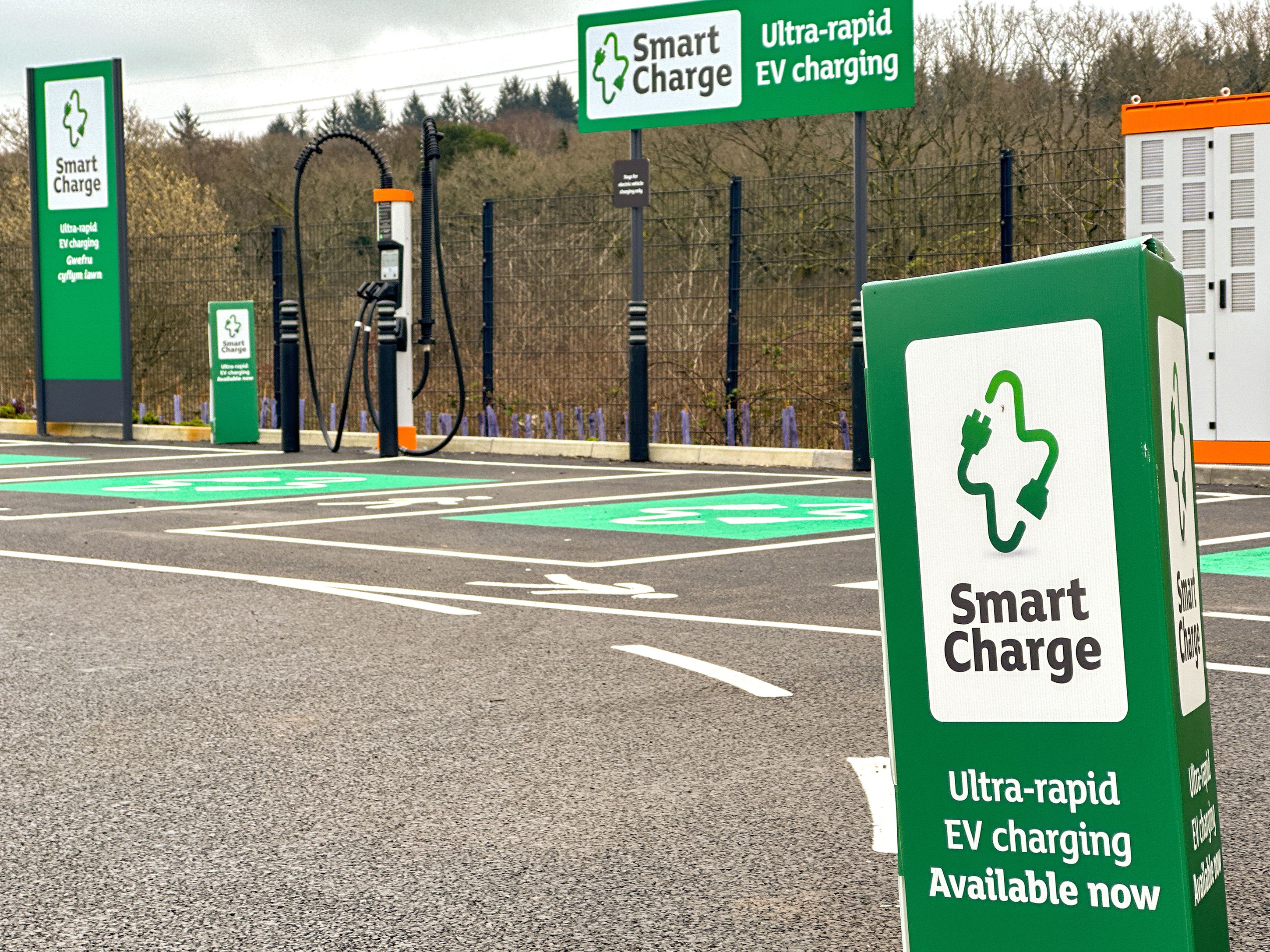
The UK government has enshrined net zero into law, but if it is to meet its targets then big strides need to be made towards decarbonising land-based transport, aviation, and shipping. Unlike most land-based transport, aviation and shipping are considered ‘difficult to decarbonise’. Their long-lived infrastructure, ongoing reliance on energy dense fuels, and issues around governance of their emissions, create particular challenges. In seeking viable and sustainable alternative fuels, hydrogen is being promoted as an option that might resolve some of the challenges around decarbonising transport, but is it really the big deal it appears to be?
Hydrogen: a suitable alternative for heavy-duty vehicles?
There are two methods to use the energy stored in hydrogen to power vehicles, namely through fuel cells or conventional internal combustion engines (ICE). Fuel cells use an electrochemical reaction between hydrogen and air across a membrane. The most common is called a PEM (proton exchange membrane) fuel cell. ICEs instead generate power through the burning of hydrogen or ammonia produced from hydrogen, in a similar way to a petrol engine. Both forms of propulsion produce water vapour as an emission, whilst ICEs also emit nitrogen oxides as byproducts.
Though produced by manufacturers, very few hydrogen-powered light duty vehicles - like cars, vans and taxis - have been sold in the UK. Instead, the preference is for electric battery-powered vehicles, particularly as costs fall and range improves. Hydrogen, on the other hand, faces poor refilling infrastructure, high fuel costs (around twice that of conventional fuel) and the inconvenience of needing large onboard storage tanks, reducing space for passengers and goods.
With electrification of private vehicles expanding rapidly, a more likely application for hydrogen is in heavy duty vehicles, such as buses and heavy goods vehicles (HGVs), either as hydrogen only or electric/hydrogen hybrids. HGVs have space for multiple storage tanks, and routes could incorporate hydrogen filling stations. Depending on fleet size, it can be feasible to install small scale hydrogen generation and storage at vehicle base stations. Indeed, hydrogen could be preferred on longer routes due to its higher range than batteries, or where vehicle downtime for battery recharging isn’t viable. However, using hydrogen in HGV fleets remains stymied by a lack of infrastructure, technology development, and costs.
Climate impacts of aviation and shipping
Shipping and aviation release a large proportion of their emissions over international airspace and waters, complicating governance arrangements. Globally, their levels of emissions are of a similar scale to a top ten emitting country and both are framed as ‘difficult to decarbonise’. Yet as our research has shown, when exploring options to cut emissions, they are different in almost every way, and in the case of shipping, perhaps not so ‘difficult to decarbonise’, after all.
When it comes to climate impacts, where in the atmosphere the emissions are released, matters. Emissions of CO2 are long-lived, becoming well mixed in the atmosphere. However, ships and planes create warming and cooling through their other pollutants, too. Measures to minimise sulphur emissions from ships by enforcing cleaner fuels near coasts are in place and likely to be strengthened; the UK government is currently consulting on the matter, but the challenge around aviation is more problematic. Aircraft release a large proportion of their emissions into sensitive regions of the atmosphere causing chemical reactions. The CO2 impacts from combusting kerosene are understood, however other emissions, including nitrous oxides, soot and water vapour have doubled the amount of climate warming from aviation, when compared with the CO2 alone. The timeframes that some of these impacts are sustained are short and geographically they do not become ‘well mixed’. This means that they haven’t been subject to policies to curb them, unlike sulphur from ships. So, any future alternative fuel choice must also minimise these harmful emissions, as well as CO2. Combustion of hydrogen would also produce water vapour and nitrous oxides, and the relative non-CO2 climate impacts are not well understood.
Mitigation measures for aviation and shipping
With both sectors needing to move away from fossil fuels, hydrogen has been gaining interest, either directly in a fuel cell or engine, or to create other fuels. Hydrogen has been muted as a ‘solution’ to decarbonising aviation for over two decades, but with its lower volumetric energy density, using it requires a redesign of aircraft to allow for a much larger fuel tank. With a slow turnover rate of the fleet, and constraints on airport infrastructure for handling aircraft of different designs, this route is unlikely to make huge inroads over the coming couple of decades. Hydrogen fuel cells for propulsion are even less likely, except for small planes, given the extra power required to uplift the fuel cells onboard.
Where hydrogen use is interesting is in creating e-fuels, a form of synthetic fuel that can be used as a fossil fuel alternative, using renewable energy. Methanol and ammonia can both be produced as e-fuels and offer benefits in terms of their energy density compared with hydrogen, although methanol requires carbon capture technology to be developed in tandem as a source of CO2. Crucially, both require sufficient renewable capacity to create the hydrogen in the first place. Furthermore, if fuel continues to be combusted in the aeroengine, the impact of other emissions released still need to be mitigated. Nevertheless, with few options to curb its CO2 emissions technologically, hydrogen will likely remain a focus.
For shipping, the picture is less clear. Although badged as ‘difficult to decarbonise’, there is already a suite of available options to cut ship emissions. Some are operational – such as slowing down or using different contracts and practice, while others, as illustrated by research from the Tyndall Centre for Climate Change Research, relate to technologies that can be retrofitted such as wing-sails, harnessing wind power and cutting fuel consumption. Even on the fuel side, with such diversity of ship sizes and types, shore-power with battery-electric propulsion is being adopted for some markets. And as it is cumulative emissions that dictate future climate change, these benefit from being able to deliver cuts now – unlike the prospect of large-scale green alternative fuels. Ammonia though, produced from green hydrogen, is a fuel of interest to the sector. Ships already transport ammonia for the fertiliser industry, and some ships are already ‘ammonia ready’ for when there is a steady green supply. Nevertheless, there are safety issues to overcome if this is to become widely adopted. Green methanol, too, is gaining interest – with some ships already combusting methanol – albeit at a small scale. It also seems unlikely that large amounts of green ammonia will be available to the industry for some time – not least because the biggest ammonia consumer – the agriculture sector, must ‘green’ its fertiliser too.
Recommendations and conclusions
Demand for hydrogen in transport is expected to increase from 1-4 TWh in 2030 to 75-165 TWh by 2050 in the UK, according to government predictions. This scenario includes use across HGVs, buses and coaches, as well as domestic and international aviation and shipping.
With a limited supply of green hydrogen currently available, it would be prudent to consider where its use is most likely and appropriate. Aviation has few technological alternatives, so could be prioritised for hydrogen use, given its limited supply. Yet with huge inequities in who uses aviation and for what, travel demand management must also be part of the picture, particularly when other sectors that are more equitably used by the population, need hydrogen too. For shipping, with its multiple options available to deliver near-term cuts, is hydrogen really the big deal it appears to be at first sight? Well perhaps in the longer term – and most likely to produce green ammonia - but scaling up production where there is a plentiful renewable resource will need to accelerate rapidly.
In light of these conclusions, the following recommendations should be considered.
- As short-term cuts in emissions fit with the climate science, the alternatives to using hydrogen that are likely to cut emissions most quickly – including demand management - should first be considered.
- Supply of green hydrogen should be prioritised for hard to abate sectors that underpin key functions of society, with electrification promoted for transport modes if technically and practically viable.
- An essential requirement to producing low carbon hydrogen is the greater availability of renewable electricity, a priority that would also support concurrent electrification.
- The UK government must revisit the previous administration’s plan for a 2024 zero emission HGV and coach infrastructure strategy to ensure it considers recent battery developments and demand, to plan any investment in refuelling infrastructure.
- Before investing in materials for storage and hydrogen transport airside at airports, who benefits from green infrastructure should be reviewed.
- Prioritise ship energy efficiency, including wind-assist retrofit, and support for shore-power, while the safety and environmental implications of using ammonia onboard ships remains poorly understood.
- Research investment is needed to find alternative hydrogen energy systems less reliant on critical rare elements, compared to current PEM fuel cells.
About the authors
Alice Larkin is a Professor in Climate Science and Energy Policy at the Tyndall Centre for Climate Change Research and is also the Energy Beacon Lead for The University of Manchester.
Amanda Lea-Langton is a Senior Lecturer in Bioenergy Engineering and lead for Environmental Energy at the Manchester Environmental Research Institute (MERI), The University of Manchester.
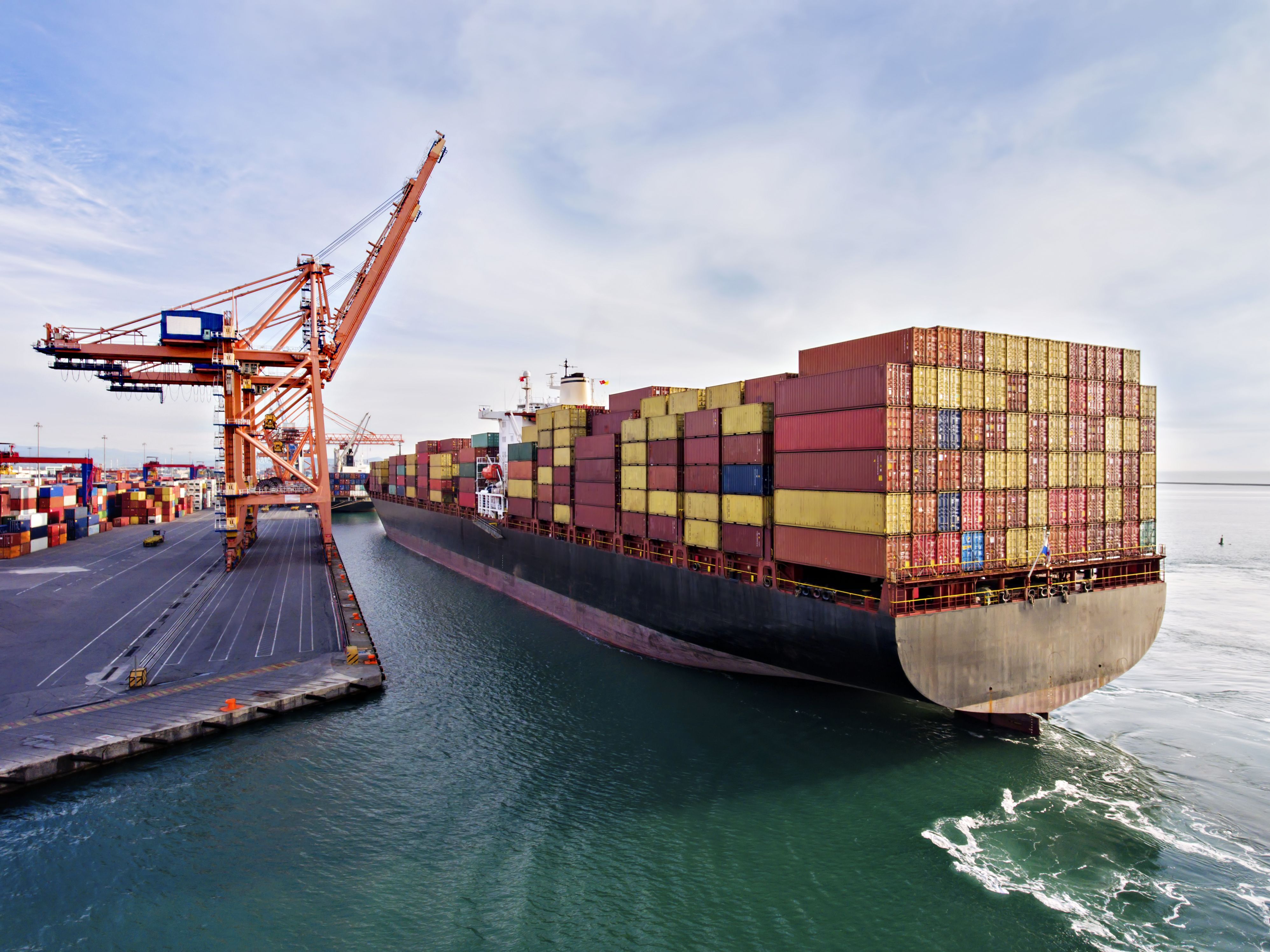
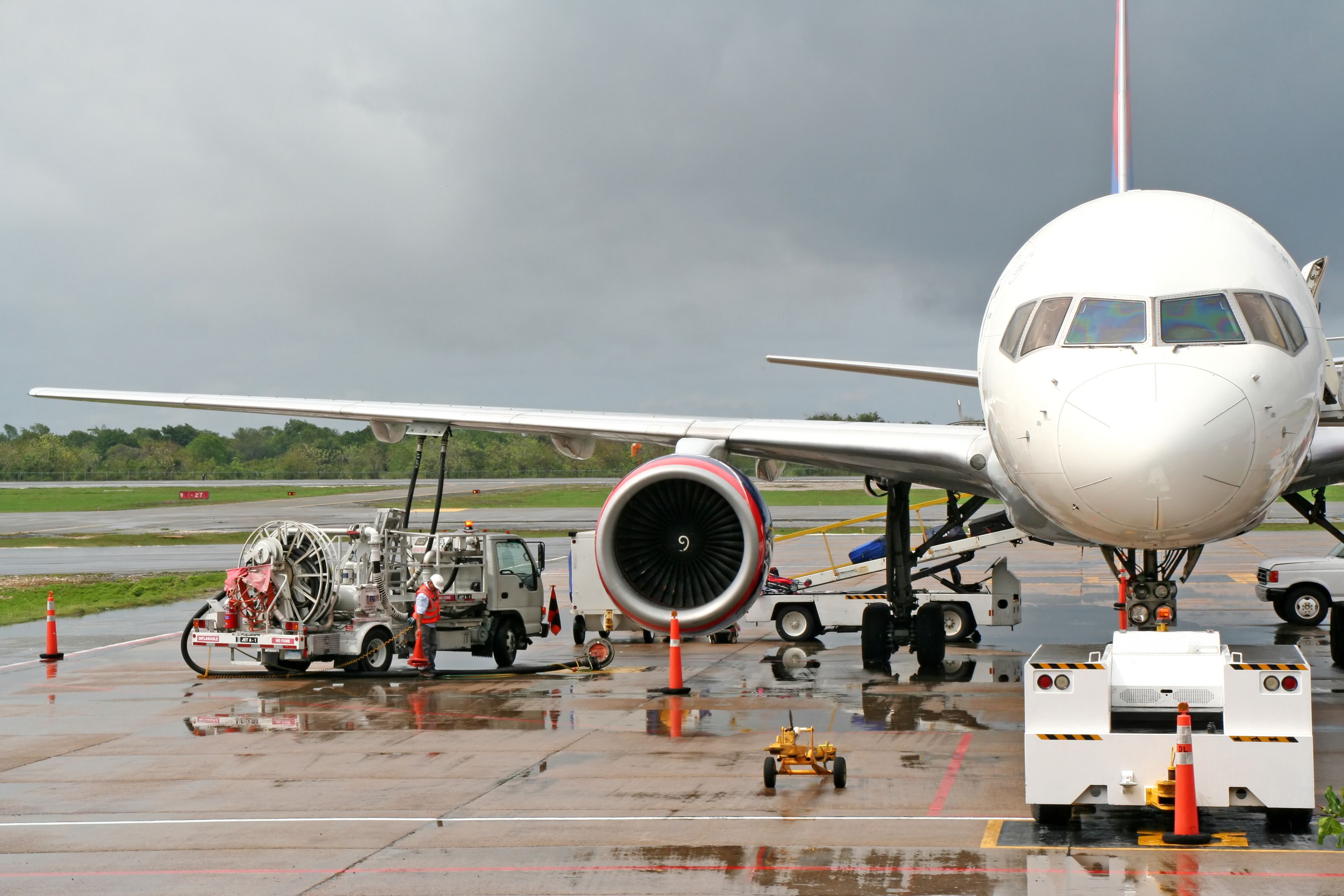
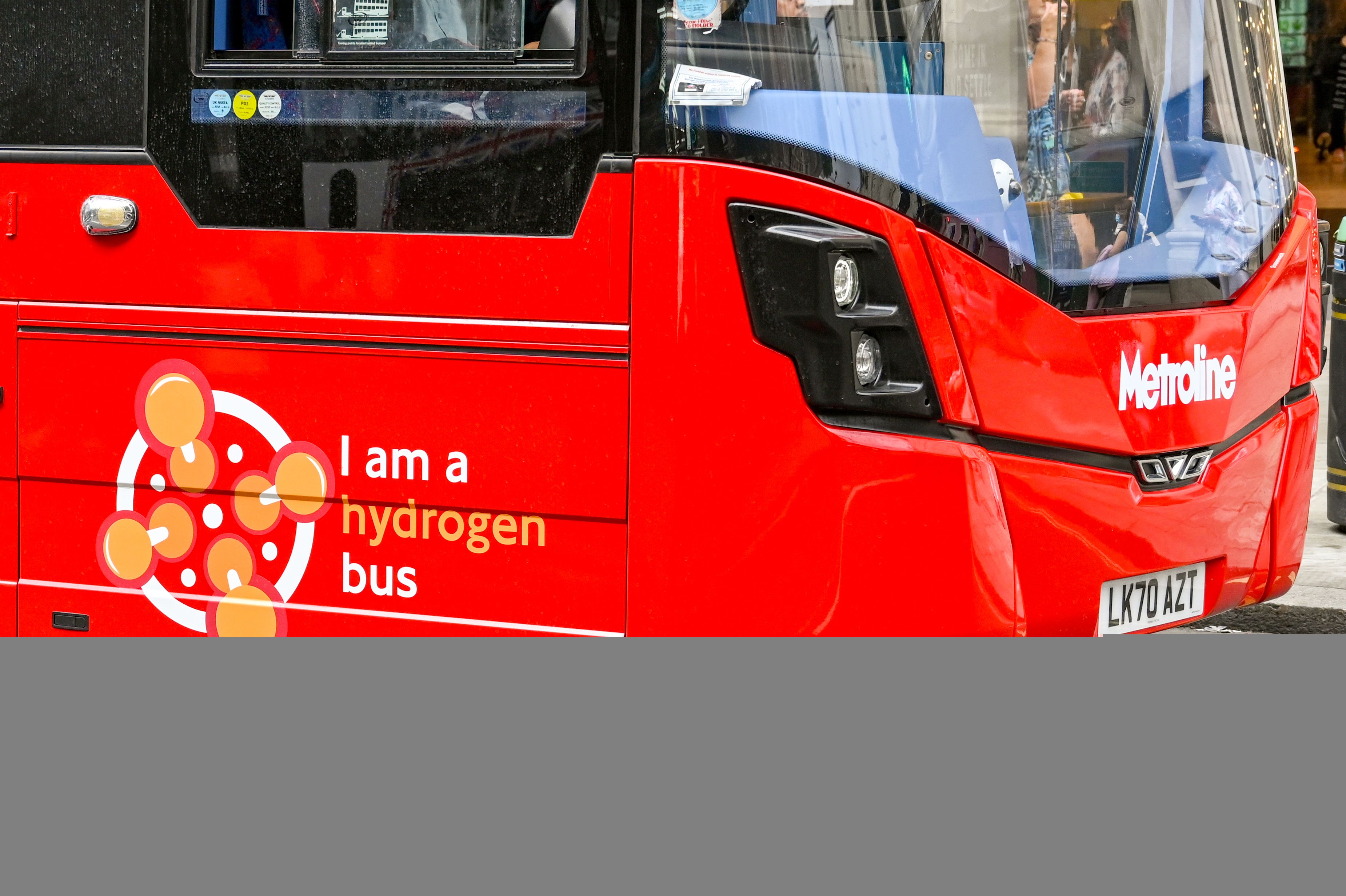
Hydrogen
the missing piece in the clean energy puzzle
Professor Aoife Foley, Dr Dlzar Al Kez, and Mr Faraedoon Ahmed
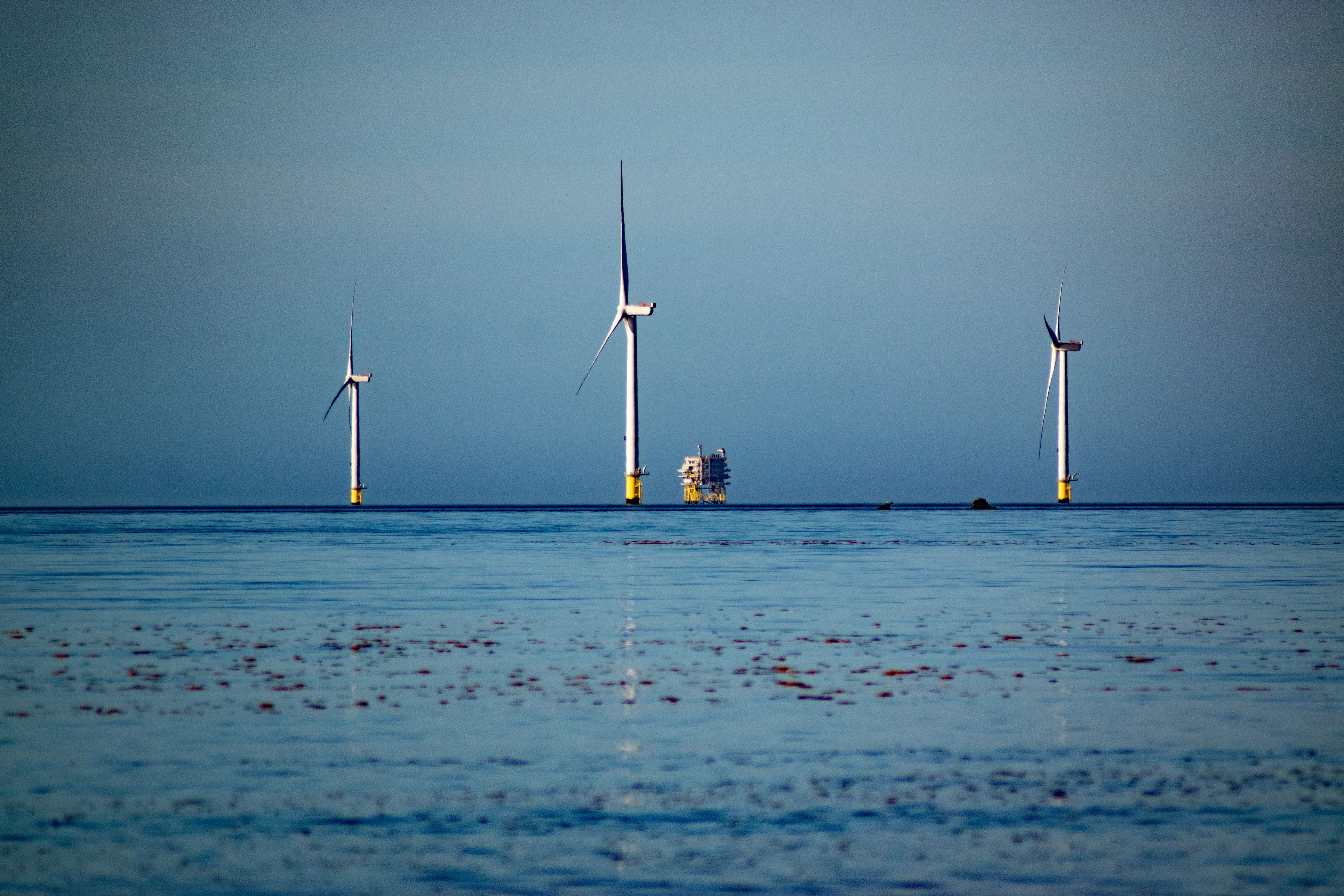
Hydrogen has been proposed as a clean energy carrier, offering a sustainable and efficient alternative to fossil fuels during the net zero transition. Hydrogen’s significance lies in its production process, which in some cases means that it can be a zero-emission energy source. Hydrogen's high energy density and versatility allow it to be used in various forms, including as a fuel for vehicles, in power generation, and as a raw material in chemical industries. This flexibility facilitates its integration into existing energy systems, making it a key player in the transition towards a cleaner and more sustainable energy future.
Hydrogen's potential
The UK's strategy to achieve its net zero emissions targets by 2050 places a significant emphasis on hydrogen as a key component of its energy mix, with ambitious targets set for hydrogen production capacity, aiming for 10 GW of low-carbon hydrogen by 2030.
Hydrogen serves as an energy vector in hard-to-decarbonise sectors such as industry, heating, and heavy transport, where alternative energy sources, like electricity, may be impractical. Its ability to offer energy storage capabilities, resilience, and potential to improve the UK's energy security by reducing dependence on imported natural gas makes it a strategic choice for the country's energy future. The UK government is actively supporting the growth of hydrogen production, distribution, and utilisation, demonstrated through initiatives like the £5.1 million RECYCLE project led on by The University of Manchester. RECYCLE aims to develop and demonstrate an innovative hydrogen production pilot unit that employs chemical looping reforming (CLR), incorporating built-in carbon capture mechanisms.
Connecting hydrogen markets and supply chains
Interconnectors (high voltage cables running across land or under the sea) serve as vital conduits for the transmission of energy between different regions and energy sources. Hydrogen interconnectors may play a crucial role in supporting the development of a global hydrogen infrastructure and can facilitate transportation of hydrogen to the grid, in order to off-set fluctuating/seasonal demand. They have several important functions.
Hydrogen interconnectors can connect UK hydrogen production and storage facilities with European and international markets, enabling the trade of hydrogen and related goods and services, aiming to meet uncertain demand. This can help support the development of the UK's hydrogen economy and international energy supply chains. Interconnectors can increase the UK's long-term energy security by enabling the import and export of hydrogen during periods of high demand or low domestic production. This flexibility can help balance supply and demand and reduce dependence on imported natural gas.
Integrating offshore wind and hydrogen production through multi-purpose interconnectors (MPIs) can create synergies and maximise the utilisation of renewable energy. Coordinated approaches to developing interconnected energy hubs in regions like the North Sea can support the development of a global hydrogen infrastructure.
Interconnectors facilitate the decarbonisation of hard-to-abate sectors, such as industry and transport, by providing a clean and scalable energy carrier. By transferring hydrogen, a cleaner fuel, from region to region, interconnectors integrate current energy markets, promote competition and price stability, and offer consumer access to a wider range of energy sources.
Interconnectors foster international collaboration in the energy sector. Cross-border infrastructure encourages cooperation between countries, facilitating the exchange of renewable energy and promoting collective efforts towards carbon neutrality.
Immense potential but many challenges
Despite its immense potential, the widespread adoption of interconnected hydrogen-electricity systems faces several challenges including technological, regulatory, and economic barriers. Enhancing the efficiency and reliability of hydrogen-electricity conversion processes is paramount. This involves advancements in electrolysis, hydrogen storage, and fuel cell technologies to ensure that hydrogen can be produced, stored, transported, and utilised effectively and efficiently.
Furthermore, significant investment is required to develop the necessary infrastructure for a hydrogen economy in the UK, including pipelines for hydrogen transportation and electrolyser capacity for hydrogen production. These investments must be carefully planned and managed to ensure they are economically viable and environmentally responsible.
Additionally, regulatory frameworks must evolve to accommodate the unique characteristics of hydrogen as an energy carrier. This includes ensuring safety standards, interoperability across different systems, and market competitiveness. Special attention must be given to cross-border energy trade regulations to facilitate fair market access for hydrogen. From an economic perspective, the cost-effectiveness of interconnector projects requires careful evaluation, alongside investment incentives and risk mitigation strategies. Continued technological innovation in electrolysis, hydrogen storage, and fuel cell technologies is crucial to drive down costs and improve efficiency.
Strategies for overcoming barriers
To fully harness the potential of interconnectors in bridging hydrogen and electricity, a strategic policy framework is essential. This framework should not only incentivise infrastructure investments but also nurture research and development, expedite certification processes, and standardise interoperability protocols. Furthermore, establishing effective market mechanisms for hydrogen trading and pricing is crucial. A fundamental element of this framework should be the introduction of incentives designed to attract private investment, spur innovation, and encourage the adoption of hydrogen technologies. These incentives could encompass tax breaks for infrastructure development, support for the research environment, simplified permitting procedures, and the offer of financial incentives for adhering to standardised protocols.
Additionally, fostering international collaboration and knowledge exchange is indispensable for driving the global shift towards a hydrogen-based economy. This entails establishing standardised pricing mechanisms to ensure transparency and consistency across international markets. Collaborative efforts should integrate markets between countries through agreements for cross-border trading and the harmonisation of regulations. Furthermore, facilitating information exchange and knowledge sharing will promote best practices in hydrogen production, distribution, and utilisation on a global scale. Such efforts will incentivise collaboration and innovation, paving the way for a more efficient and sustainable hydrogen market worldwide.
The University of Manchester, through its part in the HI-ACT Project, is instrumental in driving research towards the integration of hydrogen into future net zero energy systems. The project aims to integrate hydrogen equitably into the energy system through comprehensive, multidisciplinary research that addresses integration challenges. This aligns with the broader goals outlined in the UK's Net Zero Research and Innovation Framework Delivery Plan for 2022 to 2025.
Unlocking the benefits of hydrogen
Despite the array of challenges and barriers, the potential benefits of hydrogen-electricity integration are substantial. In order to unlock the full potential of this integration and propel the transition to a sustainable energy future, the government should prioritise interconnector infrastructure and regulation. With the support of developed infrastructure, such as interconnectors and storage, and harmonised standards, financial incentives and flexible market mechanisms can influence the demand for hydrogen.
However, this demands cross-sectoral collaborations, governments, industry stakeholders, and the engagement of research institutions, alongside long-term planning to devise and implement innovative solutions that seamlessly integrate hydrogen into the energy landscape. This would ensure both economic viability and environmental sustainability, while enhancing energy security.
Lastly, we need to be vigilant that hydrogen needs to be environmentally friendly, and that it is used for the right applications and in the right place.
About the authors
Aoife Foley is Professor and Chair in Net Zero Infrastructure at The University of Manchester.
Dlzar Al Kez is a Research Associate in Net Zero Infrastructure at The University of Manchester.
Faraedoon Ahmed is an Early Career Researcher at The University of Manchester.
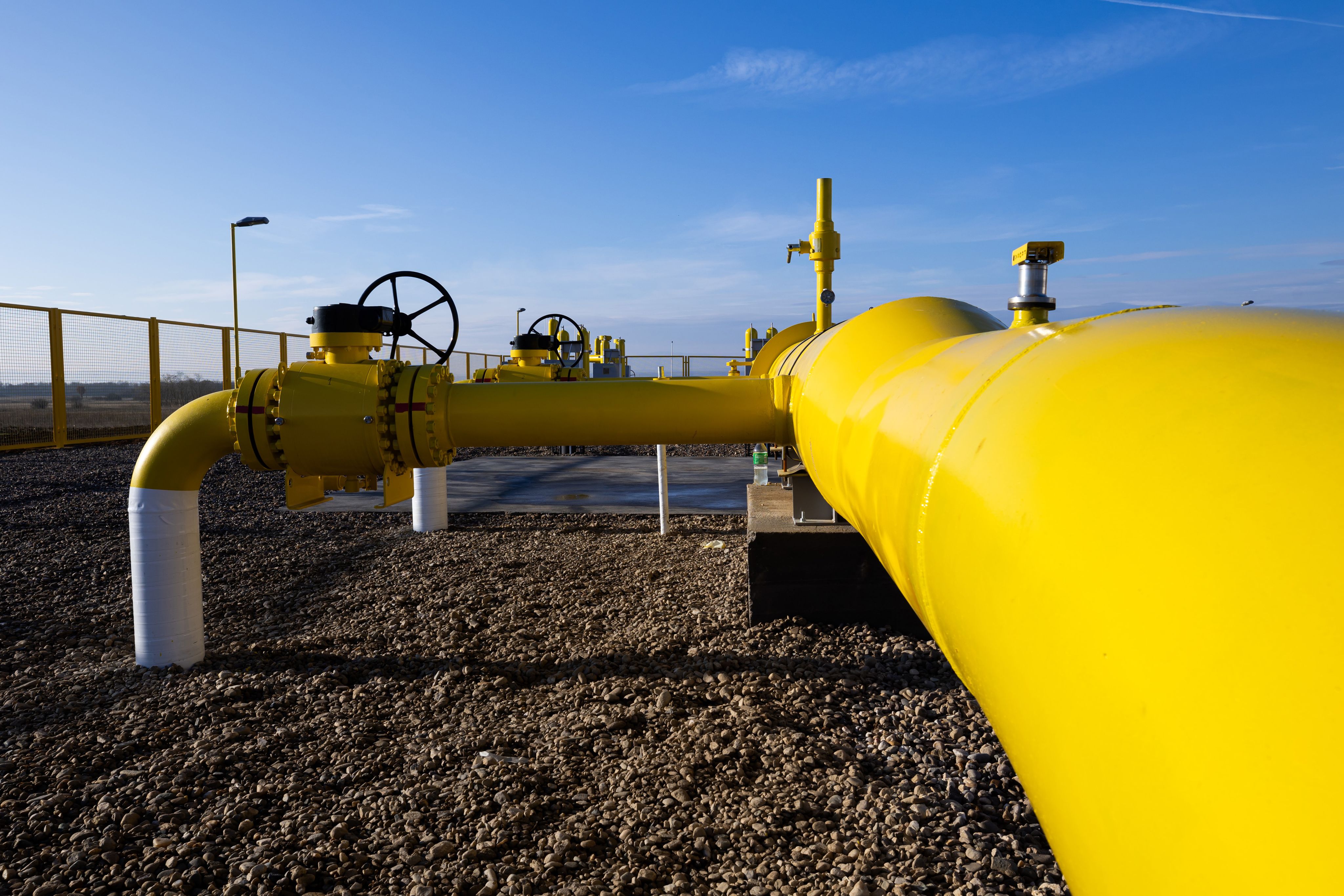
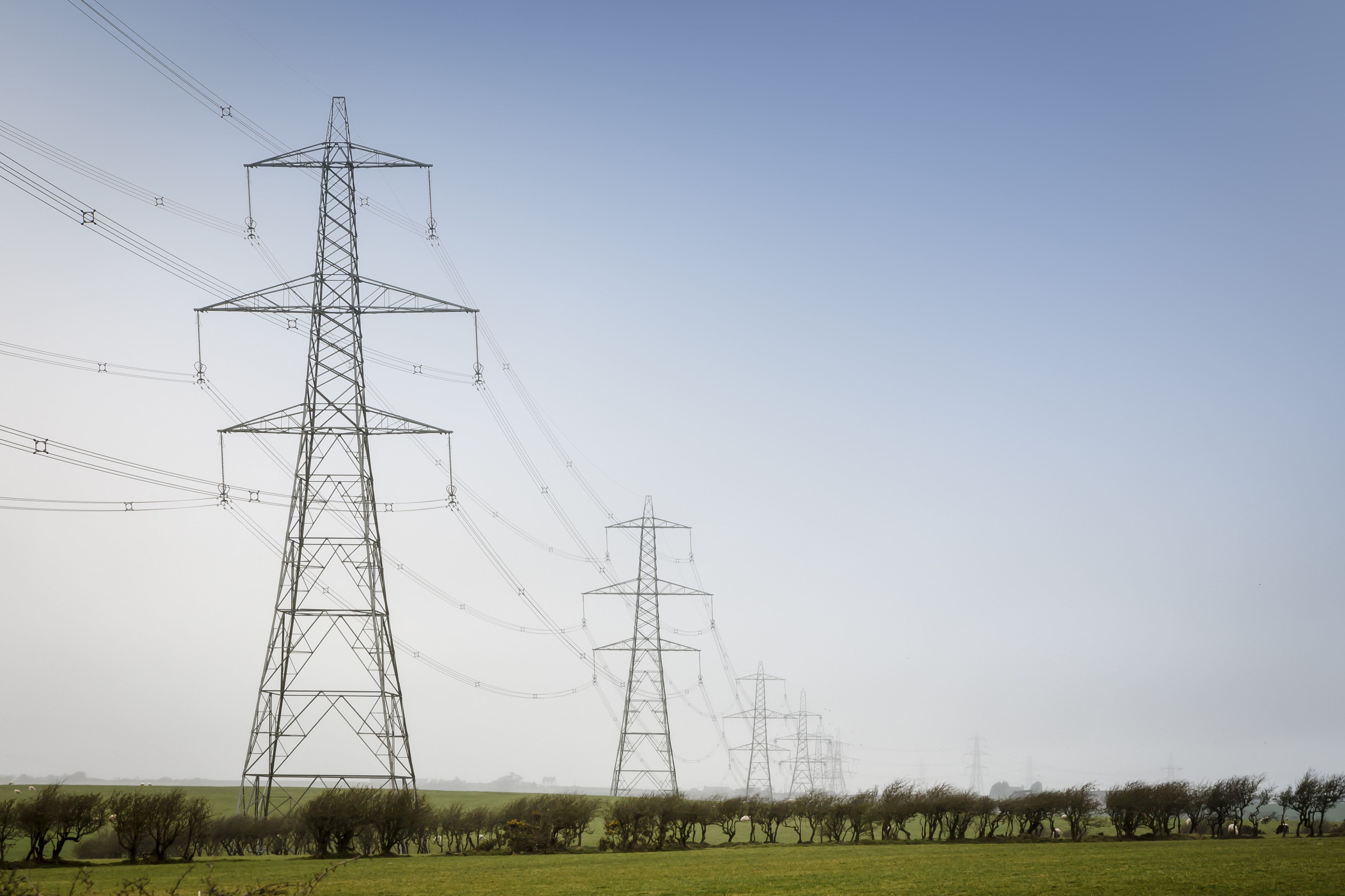
Can hydrogen help decarbonise small industrial emitters?
Dr Vincenzo Spallina and Professor Maria Sharmina
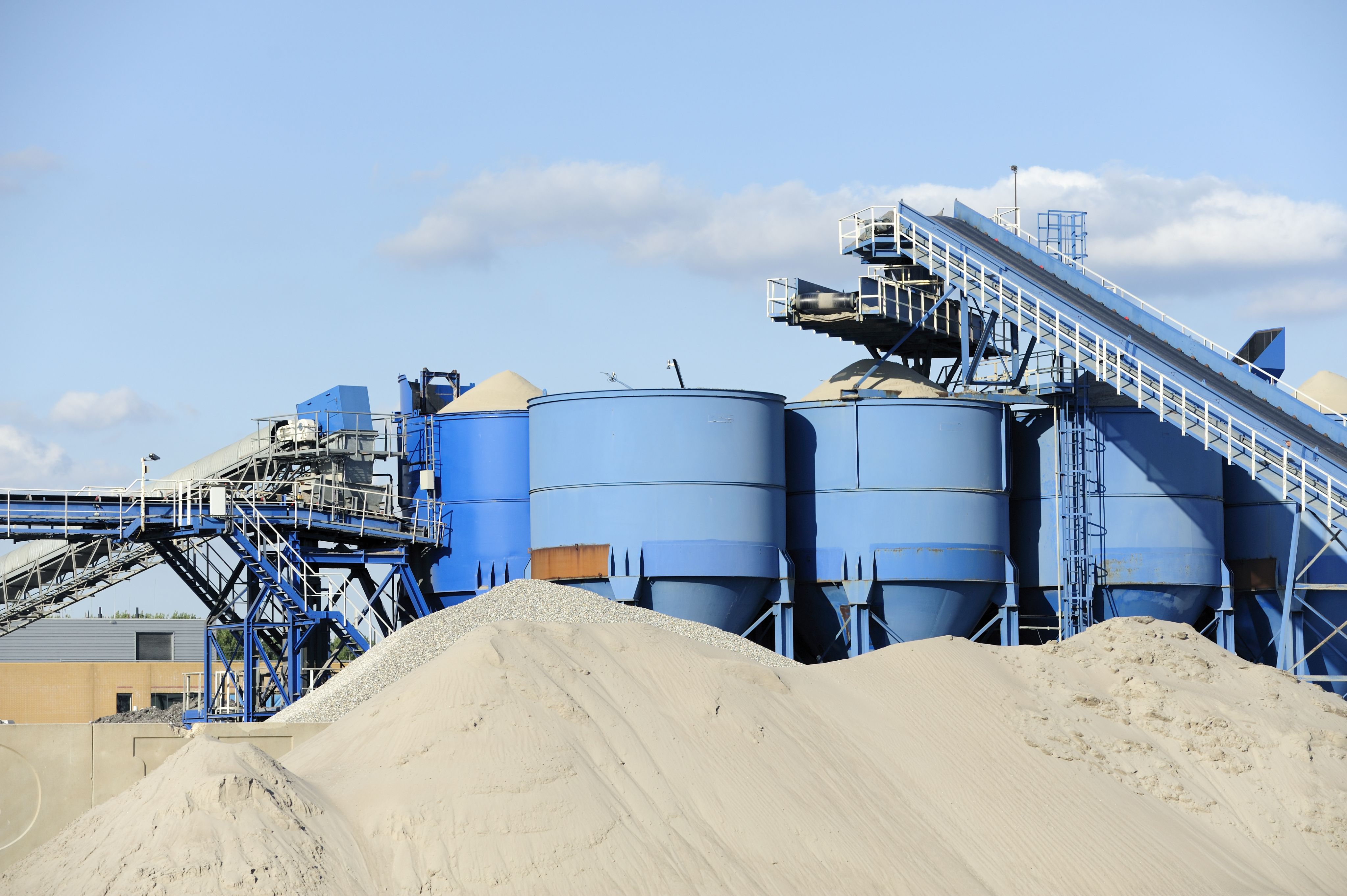
Decarbonising industry, among other hard-to-abate sectors, is a high priority in the UK as the country embarks on a pathway to net zero greenhouse gas emissions by 2050. The government’s focus to date has been on the decarbonisation of large-scale energy-intensive industries. However, more than a fifth of the UK’s carbon dioxide emissions come from small and medium-scale industrial emitters who face an uphill struggle in their transition to net zero.
Who are small industrial emitters?
Small industrial emitters are widely distributed across the country, sometimes remotely located, and their businesses span chemical, mineral, cement and lime, food and the commercial and public administration sectors. In each case, their annual emissions are usually under 150,000 tonnes of carbon dioxide.
Some of those emitters can reduce their energy demand through circular economy principles such as reuse of waste products and waste energy recovery, which could be encouraged by the government through awareness campaigns and reduced UK ETS (Emission Trading Scheme) carbon pricing. However, others will need energy supply focused solutions to support their efforts to decarbonise.
How can small-scale emitters decarbonise?
Small industrial emitters face several barriers to reducing their emissions, including cost, limited in-house expertise, and safety concerns associated with the process’s complexity. For emitters not located in major industrial clusters, transporting carbon dioxide could mean prohibitive costs to pay for ground piping or road transportation.
Options for small industrial emitters include renewable electrification, hydrogen, and biomass-to-energy. Technical viability, geographic availability and the wider environmental impacts of these options will mean they are not suitable for all businesses.
For emitters that require primarily low-temperature heat, electrification could be the preferred option. However, renewable electricity needs infrastructure for production, transmission and distribution.
Work undertaken as part of the RECYCLE project at The University of Manchester demonstrates that there is great potential for low carbon hydrogen in the UK, with implications for industrial stakeholders, including small-scale hydrogen end users. While this pilot is ongoing, it is thought that where electrification is not possible, the only viable options to decarbonise those industrial emitters would be through either hydrogen from biomass or carbon capture and storage (CCS).
CCS refers to the removal of carbon dioxide from industrial processes so that gases are not released into the atmosphere. The captured carbon dioxide is of high purity and can be sent for permanent storage or used in other chemical process. In the UK, the target is to capture at least 70 million tonnes annually by 2050, if we are to meet the Paris Agreement goals, primarily in the recently established industrial clusters across the country.
Production of hydrogen by using electrolysers at the gigawatt (GW) scale faces challenges similar to renewable electricity, as it will require a larger electrical grid than we have currently. What’s more, continuous production of electricity-based hydrogen would need additional investment in energy storage because renewable energy varies with time and season.
Supporting the transition to net zero
To make the transition affordable for small industrial emitters, technological and infrastructure measures will need to be facilitated by public policies. Such measures include: implementation of non-electric production of hydrogen; retrofitting of small industrial emitters to accommodate carbon capture; and coordination of transport for both hydrogen feedstock and carbon dioxide.
Non-electric hydrogen production, such as biomass gasification and waste-to-hydrogen plants, can be carbon-neutral thanks to using biomass as a feedstock. In addition, small-to medium-scale blue (i.e. natural gas based) hydrogen production can provide energy for those emitters that cannot transport carbon dioxide to large CCS clusters. Both options would reduce the risk of intermittent hydrogen, relieve the burden on the electrical grid, and can operate in the medium term before other low-carbon infrastructures are deployed at scale.
To complement non-electric hydrogen, strategically located medium scale ‘carbon hubs’ are needed to coordinate taking captured carbon dioxide from small industrial emitters and transporting it to large CCS clusters. Any transportation itself would need to be low-carbon, for example through hydrogen-based heavy goods vehicles.
Government and relevant local authorities could co-fund retrofits for the place-based manufacturing processes, while a third party would collect and transport purified carbon dioxide. This arrangement would be similar to how local authorities deal with municipal waste by outsourcing its collection and processing to specialist waste management firms. The funding could come from the ringfenced carbon levy collected through the UK’s carbon border adjustment mechanism.
To optimise infrastructures and resources (e.g. electricity, hydrogen, and biomass) for the medium-scale carbon hubs, locations of small industrial emitters, electricity transmission and distribution, and transport links would need to be mapped. Such a mapping exercise could be co-funded by the Government either through UK research councils or through a tender.
Policy recommendations
Several policies would speed up the decarbonisation of small industrial emitters:
- Expand the Industrial Decarbonisation Challenge competitions to include deployment and cluster plans for small industrial emitters.
- Require the recently established large industrial clusters to attract, train and host experts, tasked with providing small industrial emitters with expertise in decarbonisation and related safety matters.
- Bring forward the implementation of the carbon border adjustment mechanism from 2027 to 2025, to level the playing field with imported manufactured goods sooner. The carbon levy can be phased in early, starting with the most carbon-intensive goods only (such as steel) and expanding the list of goods each year.
- Fund research and modelling to compare potential electricity-based vs. hydrogen-based and carbon-capture supply chains for decarbonising small industrial emitters.
- Aligned with the carbon levy, introduce progressively tightening emission standards for heavy goods vehicles within these supply chains, to avoid carbon emissions from transportation.
- Reduce UK ETS (Emission Trading Scheme) carbon price for reused and recycled industrial inputs, as part of encouraging a circular economy approach. Any reuse and recycling should be accompanied by life-cycle assessment to ensure that reuse processes themselves do not unintentionally increase carbon emissions.
- Sponsor a large-scale public and business awareness campaign about maintaining, repairing and the durability of goods (calling out wasteful practices like planned obsolescence), thereby reducing demand for industrial production.
About the authors
Vincenzo Spallina is a Reader in Chemical Engineering in the School of Engineering at The University of Manchester.
Maria Sharmina is Professor in Energy and Sustainability at the Tyndall Centre for Climate Change Research in the School of Engineering at The University of Manchester.
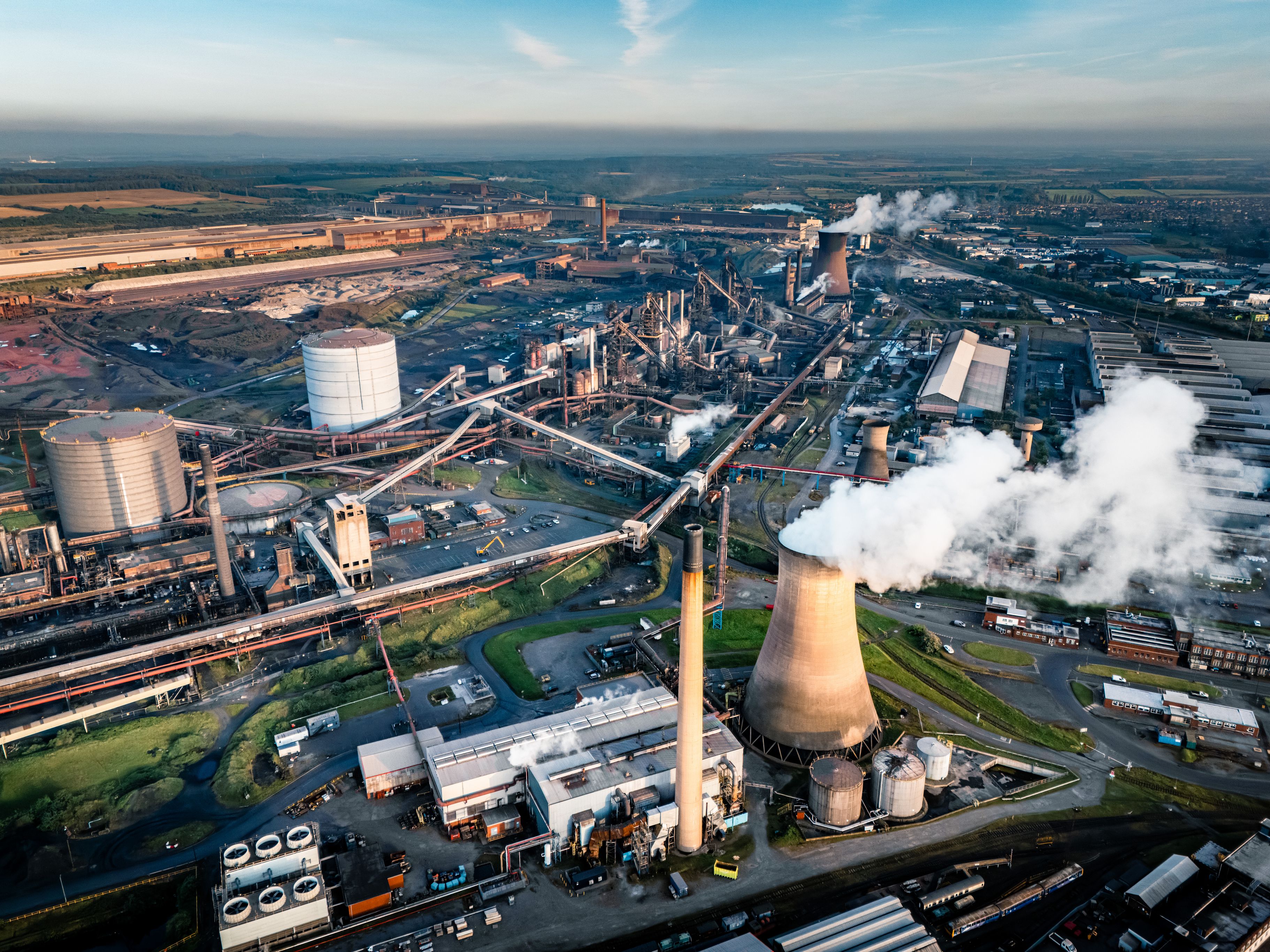
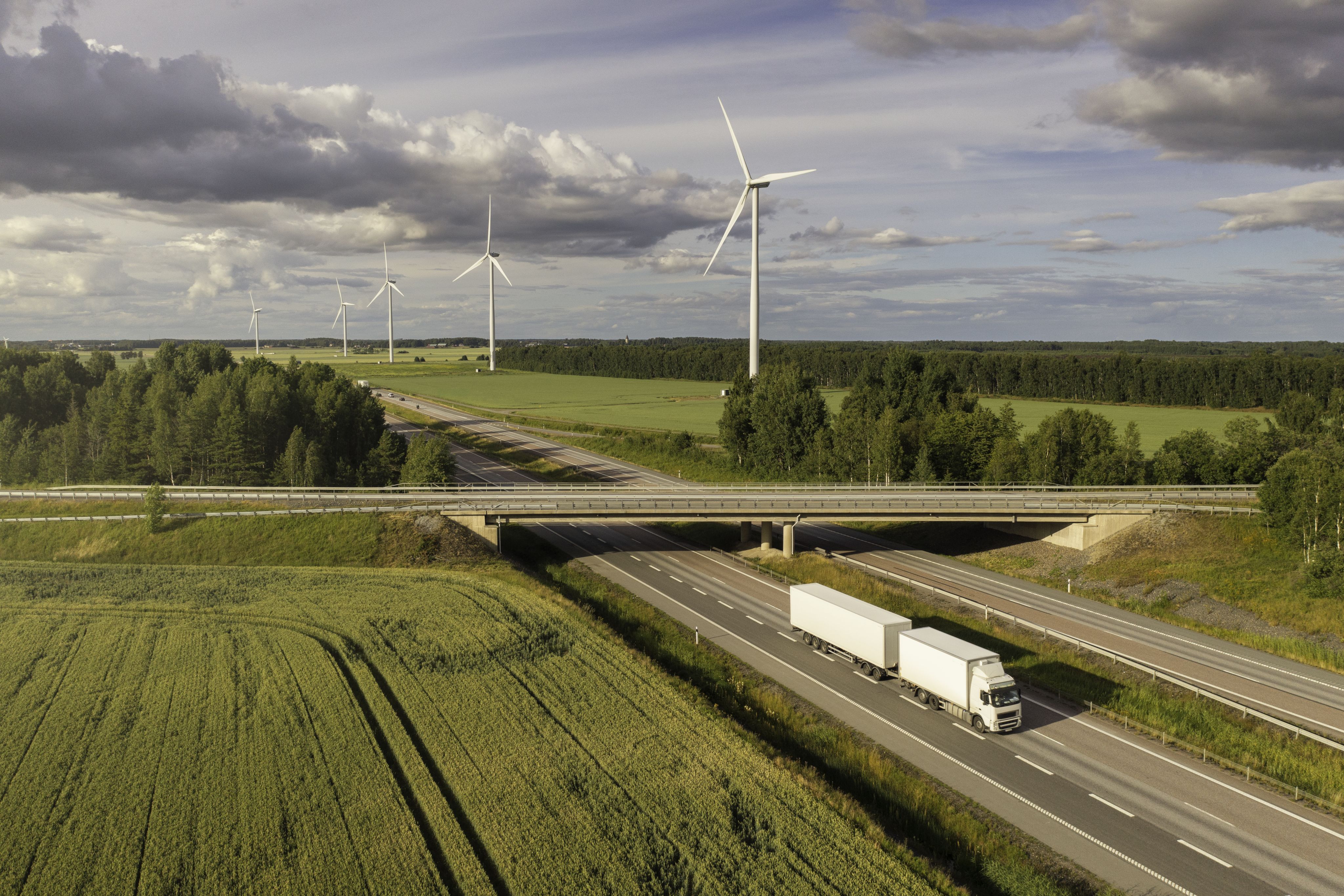
With special thanks to our contributors:
Faraedoon Ahmed is an Early Career Researcher at The University of Manchester.
Dlzar Al Kez is a Research Associate in Net Zero Infrastructure at The University of Manchester.
Will Bodel is a Fellow in Nuclear Energy Policy at The Dalton Nuclear Institute, The University of Manchester.
Aoife Foley is Professor and Chair in Net Zero Infrastructure at The University of Manchester.
Christopher Jones is a Senior Knowledge Exchange Fellow at the Tyndall Centre for Climate Change Research, The University of Manchester.
Alice Larkin is a Professor in Climate Science and Energy Policy at the Tyndall Centre for Climate Change Research and is also the Energy Beacon Lead for The University of Manchester.
Amanda Lea-Langton is a Senior Lecturer in Bioenergy Engineering and lead for Environmental Energy at the Manchester Environmental Research Institute (MERI), The University of Manchester.
Lin Ma is a Senior Lecturer in Chemical Engineering in the School of Engineering at The University of Manchester.
Maria Sharmina is Professor in Energy and Sustainability at the Tyndall Centre for Climate Change Research in the School of Engineering at The University of Manchester.
Kevin Taylor is a Professor of Geoscience in the Department of Earth and Environmental Sciences at The University of Manchester.
Vincenzo Spallina is a Reader in Chemical Engineering in the School of Engineering at The University of Manchester.
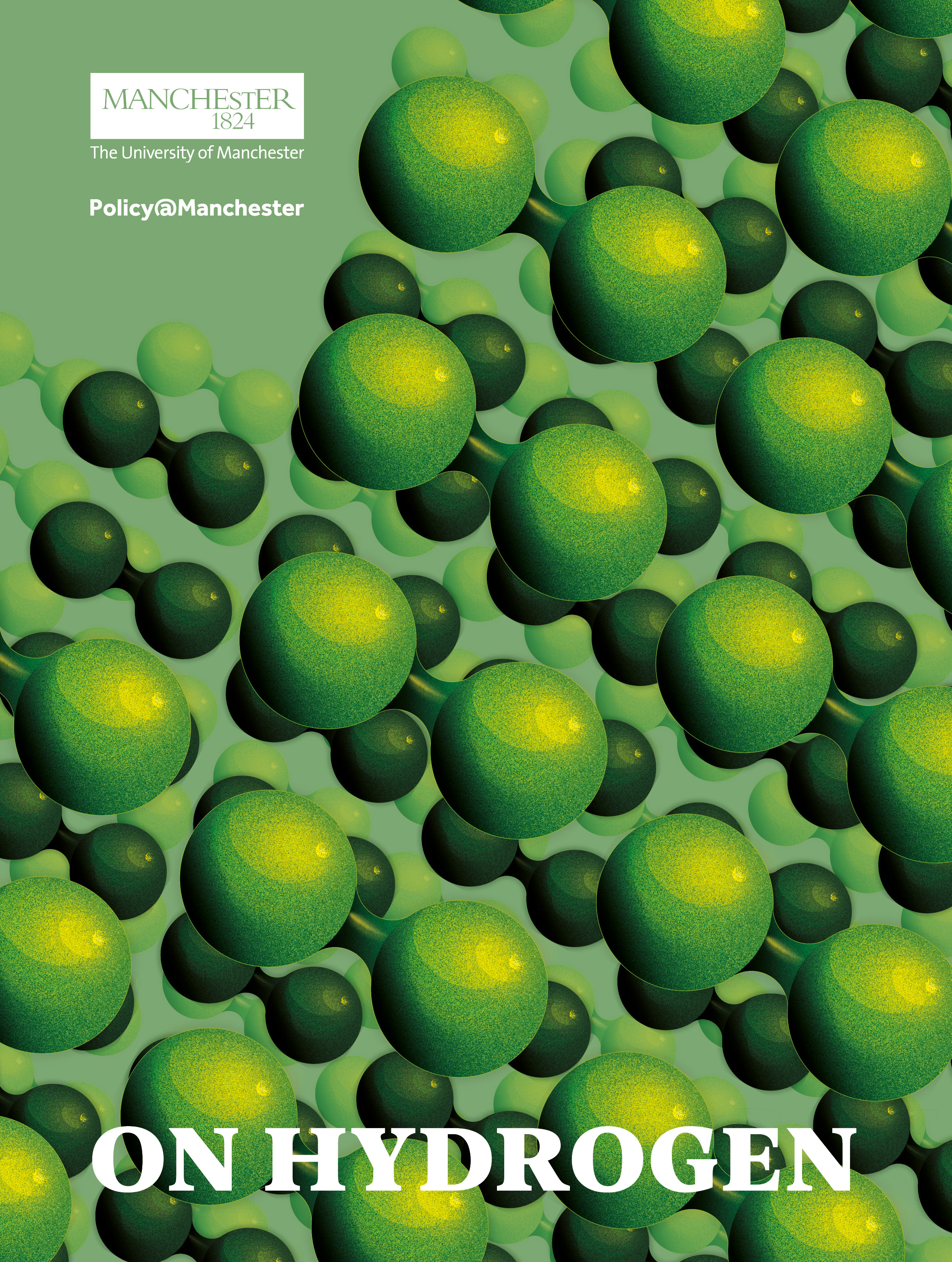
Thought leadership and ideas on hydrogen
Curated by Policy@Manchester
Read more and join the debate at blog.policy.manchester.ac.uk
#OnHydrogen
The University of Manchester
Oxford Road
Manchester M13 9PL
United Kingdom
The opinions and views expressed in this publication are those of the respective authors and do not necessarily reflect the views of The University of Manchester. Recommendations are based on authors’ research evidence and experience in their fields. Evidence and further discussion can be obtained by correspondence with the authors; please contact policy@manchester.ac.uk in the first instance.
December 2024