Carbon Capture and Storage
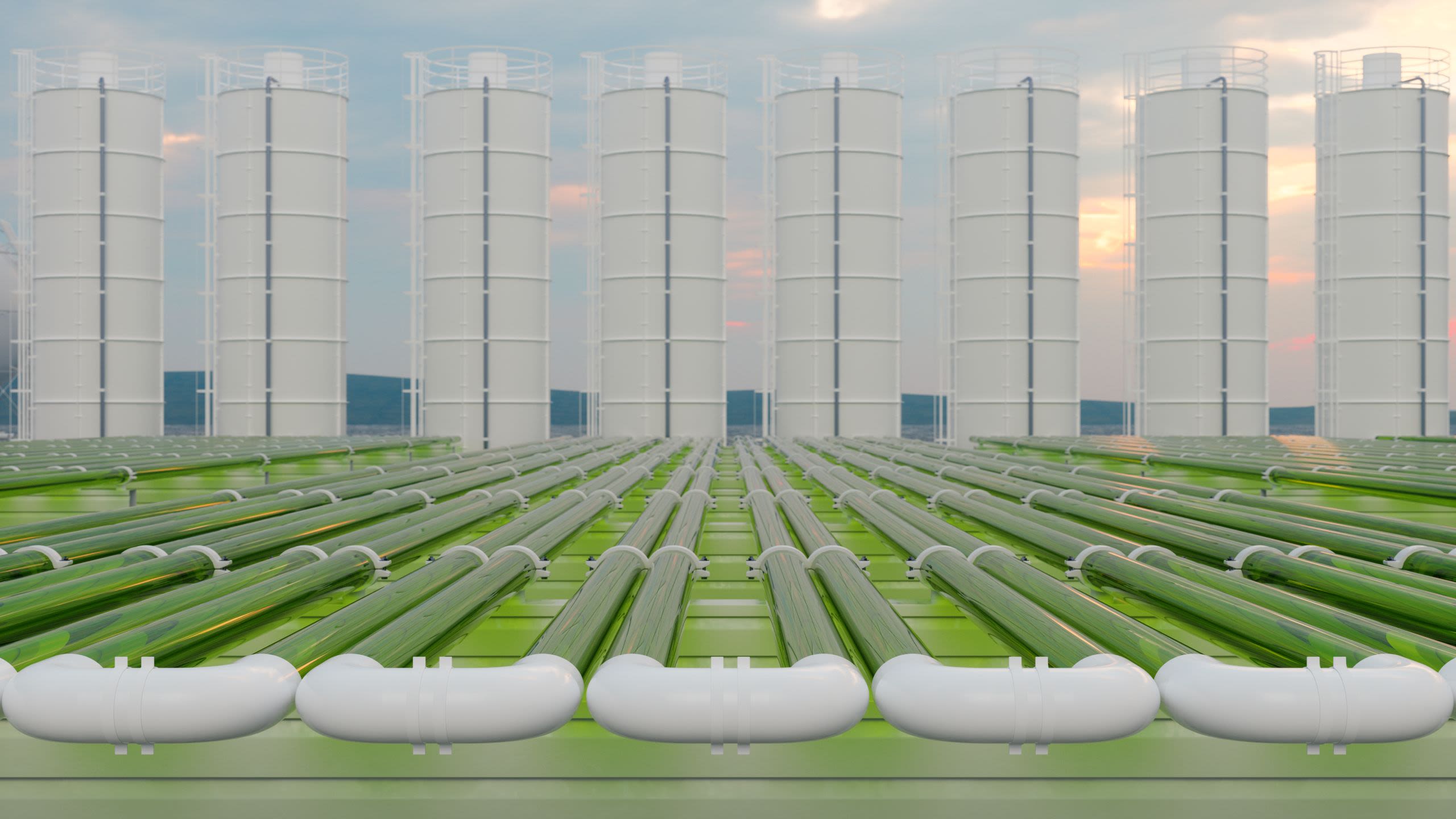
Carbon Capture and Storage and Greenhouse Gas Removal – Essential ingredients for net zero
Sarah Mander and Clair Gough
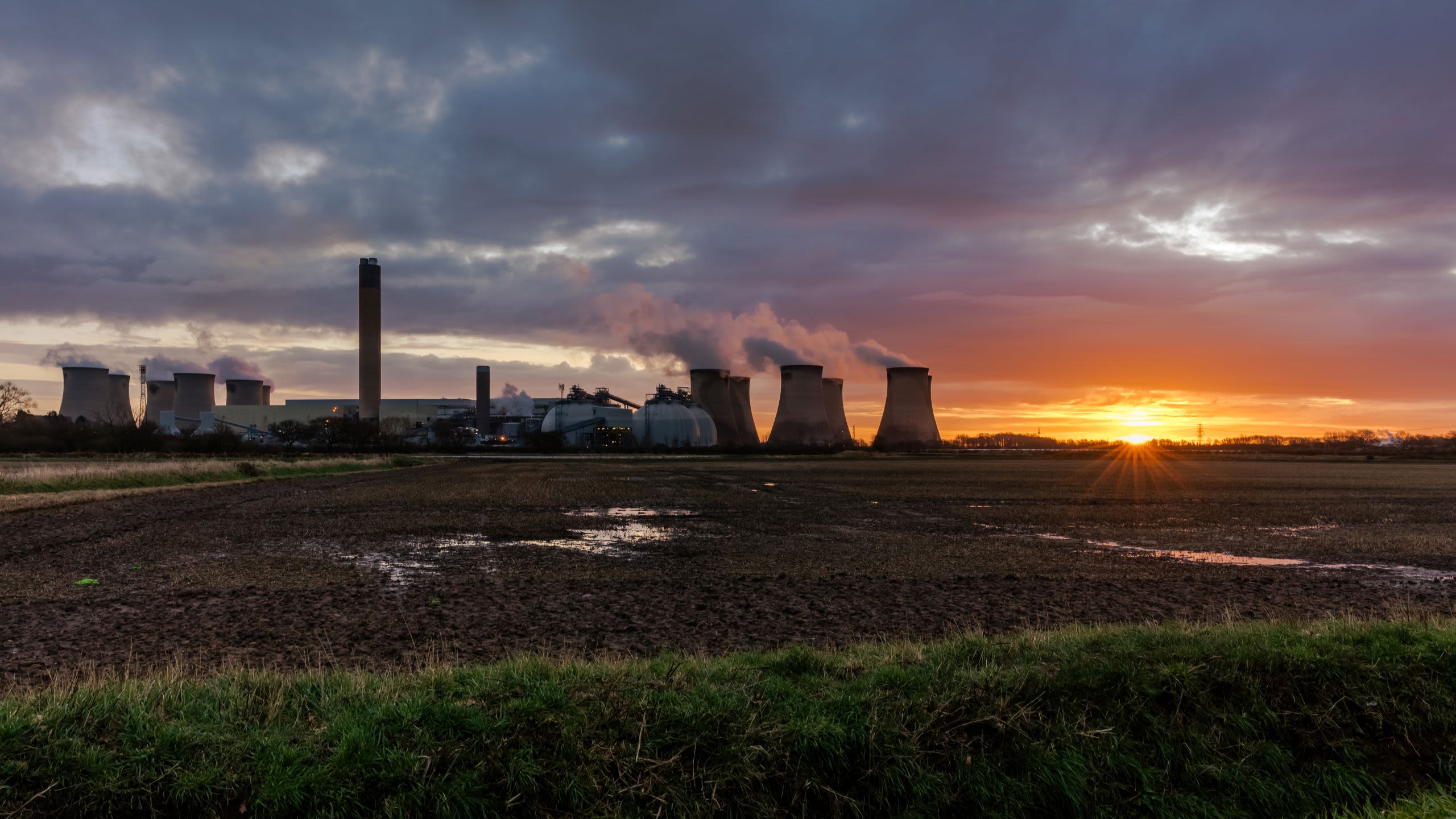
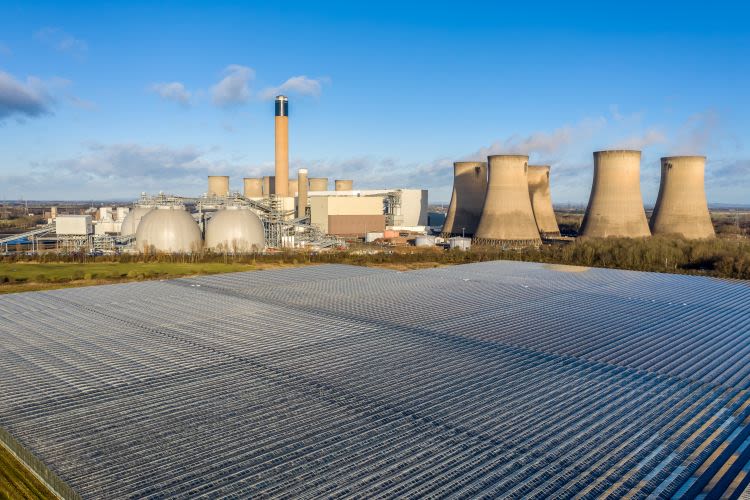
Our planet is heating up, and the impacts of climate change are already being felt across the world. We all know reducing greenhouse gas emissions is essential for our planet’s future. However, research shows that, given the scale and urgency of the challenge of reducing emissions across the entire economy, meeting climate change targets will require both carbon capture and storage and greenhouse gas removals. In this blog, Dr Clair Gough and Dr Sarah Mander discuss the necessity for carbon capture and storage in tandem with greenhouse gas removals to achieve the UK’s net zero ambitions.
- Reaching net zero, whilst retaining a thriving industrial sector, requires carbon capture, both within and beyond industrial clusters, and access to shared transport and storage infrastructure.
- Achieving the changes required to deliver net zero will require approaches with a strong social licence to operate and place-based planning processes which are just.
- Cross-sectoral decarbonisation requires a strategic approach, including prioritising use of hydrogen and biomass in the sectors where they are most effective.
CCS is essential for decarbonising industry
To meet the UK’s net zero ambitions there is a legal target to reduce greenhouse gas emissions by 78% by 2035 compared to 1990 levels, as set out in the Climate Change Committee’s (CCC) Sixth Carbon Budget. Many industries emit CO2 through fossil fuel use, while others, such as steel, cement and some chemical production, emit CO2 directly during production. Carbon Capture and Storage (CCS) is the capturing, transporting and long-term disposal of CO2. Without CCS, it will be impossible to bring emissions from certain industries close to zero, even with improved efficiency and circular economy approaches.
2022: a critical year for climate policy action
The CCC recommend 22Mt of CO2 per year is captured and stored in 2030, but policy support for CCS over the past decade has been intermittent. However, with the Ten Point Plan for a Green Industrial Revolution in 2020 and the UK Net Zero Strategy, policy ambitions for CCS of 20–30Mt CO2 captured per year by 2030 from four clusters, are now aligned with the CCC recommendations. Government strategy and funding alongside plans within the clusters for developing CO2 transport and storage infrastructure, suggest progress towards deployment by 2025. 2022 will be a crucial year for CCS as investment decisions will be made for two clusters, HyNet NW and the East Coast Cluster, which have been selected to bid for Track 1 funding under the £1billion government CCS Infrastructure Fund, which is intended to provide the certainty to secure private investment for widescale deployment of CCS.
Decarbonising industry within and beyond the clusters
The industrial clusters host a concentration of high emitting industries and the majority are in locations with access to offshore geological storage sites. Each cluster is unique in terms of its configuration and strategy for decarbonisation, although production of hydrogen features heavily in all cluster plans. Although current policy focus is on six clusters, the significant industrial emissions outside of the clusters cannot be ignored. Developing CCS infrastructure extensive enough to be accessed by multiple emitters is crucial for delivering net zero by enabling decarbonisation across multiple sectors, to provide emissions abatement and greenhouse gas removal. Research undertaken at The University of Manchester, as part of the UK CCS Research Centre and the new Industrial Decarbonisation Research and Innovation Centre, is exploring how the clusters can develop and deploy CCS with a strong social licence to operate (SLO) as part of a “just transition” which is fair and sustainable to a net zero world.
Social Licence to Operate and Just Transitions
Industrial decarbonisation entails a variety of technologies applied across different industries and extends across multiple supply chains. These socio-technical systems involve actors from industrial, regulatory and governance sectors as well as communities living close to, and working within the industrial cluster. Alignment of priorities and a shared understanding of the changes required to deliver net zero will require approaches that are seen as credible solutions and that how they are delivered, managed and regulated is trusted. This is known as a social licence to operate (SLO). Research at The University of Manchester is looking at how the SLO is developing in the clusters and the opportunities for building a strong SLO for industrial decarbonisation. The Research is also focusing on how capability for social and environmental justice for communities and workers can be included within place-based planning.
Importance of common shared infrastructure and economies of scale
If CO2 is to be stored for the long term, emitters require access to transport and storage. Initially this will be developed in the clusters, where the geographical proximity of emitters and access to offshore storage locations offer economies of scale, reducing the initial costs of deployment. In addition to CCS for abatement of CO2, a variety of potential greenhouse gas removal approaches are proposed, some of which depend on access to CCS infrastructure, for example bioenergy with CCS or Direct Air CCS. These provide opportunities for permanent removals with secure storage. Research is modelling the carbon implications of different bioenergy with CCS supply chain locations and feedstocks to understand how infrastructure and resources can be used effectively.
A strategic approach
The transition to net-zero is a challenge that cuts across sectors and energy vectors. Cross-sectoral decarbonisation requires a strategic approach, including prioritising use of hydrogen and biomass in the sectors where it can reduce carbon emissions most effectively. While ambitions for an expanding hydrogen economy grow, there is currently limited hydrogen production in the UK, and current production methods are not low carbon. Each of the industrial clusters include low carbon hydrogen production in their plans. There are multiple challenges and trade-offs to greenhouse gas removal approaches and biophysical and societal limits to scale. Greenhouse gas removal cannot be used to offset unlimited emissions, it needs to be used in tandem with other prioritised approaches to bring emissions closer to zero.
Insight for policymakers
- Developing CCS infrastructure is crucial for delivering net zero, where it can play a role across multiple sectors.
- CCS needs to be part of a strategy to reduce emissions across the whole economy, aligned with the socio-technical configurations of industries to determine what technologies are used and where they are used, including consideration of supply chains, in particular for delivering bioenergy with CCS.
- CCS development should sit alongside detailed strategy for improving efficiency and reducing material consumption in manufacturing and construction.
- Spatially explicit analysis is required to ensure a strategy is appropriate to different contexts, and recognises the importance of emissions along supply chains.
- Reaching net zero by 2050 depends not just on establishing the industrial clusters but ensuring emissions outside the clusters are included in the strategy.
- It is necessary to develop the detail behind the high-level strategy statements and create a concrete plan for delivery, to provide industry confidence in investment and innovation.
Dr Sarah Mander is a Reader and theme coordinator for Behaviour and Governance at the Tyndall Centre at The University of Manchester. Her research is focused on climate change mitigation and she has researched renewable energy, long term energy scenarios, sustainable energy in the urban environment, recycling and climate change governance.
Dr Clair Gough is a Senior Research Fellow with the Tyndall Centre for Climate Change Research. Her research has integrated technical and social science analyses in the context of energy and climate change, focusing on the assessment of carbon dioxide capture and storage (CCS), including the use of biomass energy with CCS (BECCS).
How to incentivise bioenergy with carbon capture and storage responsibly
Rob Bellamy
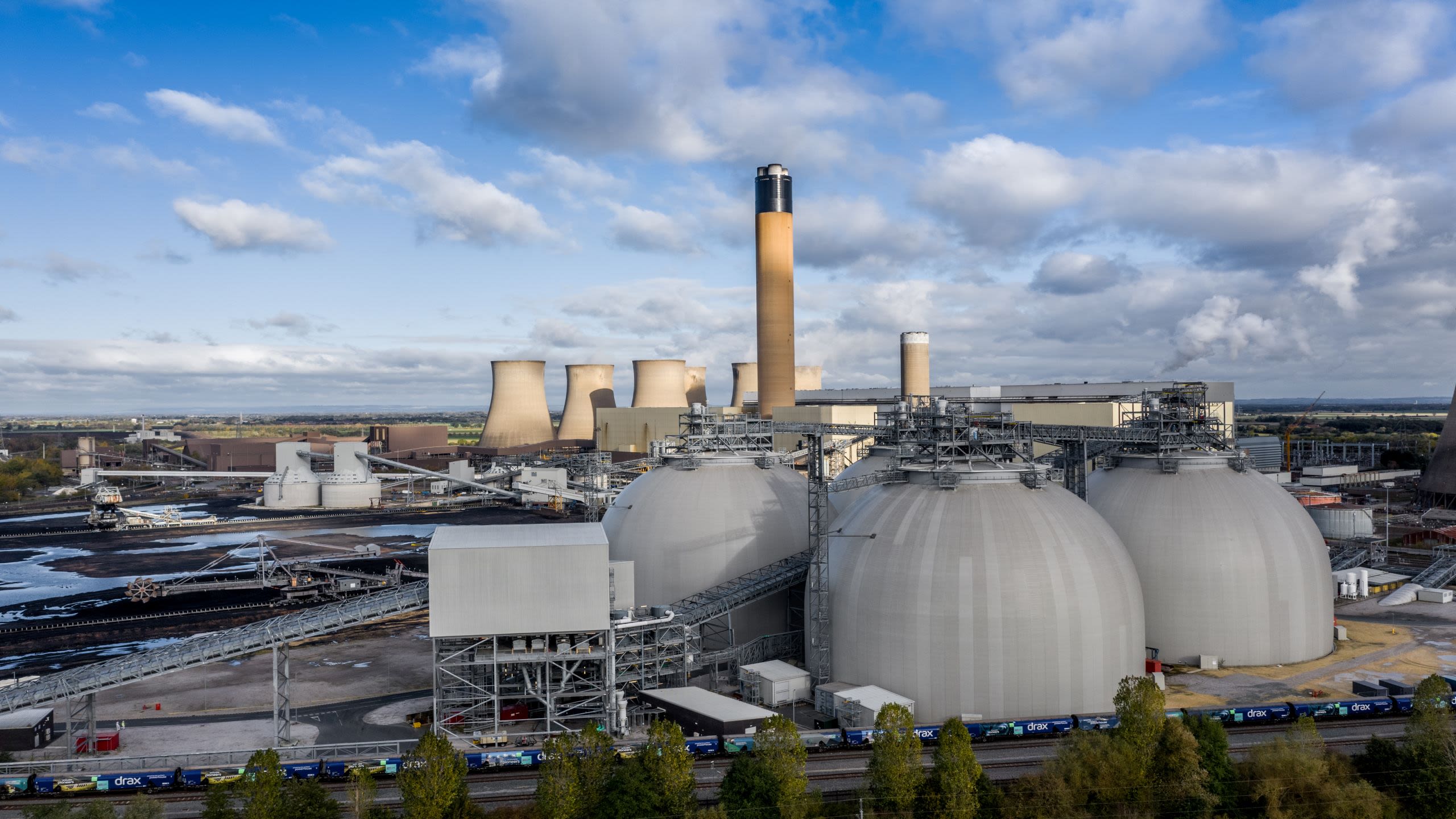
The latest IPCC report on the state of the world’s climate shows that the remaining carbon budget – the amount of CO2 that can still be emitted while keeping global warming below 1.5 degrees – is almost gone. To meet net zero within these parameters, we will need to remove CO2 that is already in the atmosphere. One method of achieving this is by using bioenergy with carbon capture and storage (BECCS). However, BECCS is not yet ready for use at scale, and raises societal concerns around land use for biomass, the safety of stored carbon, and potential distraction from emissions reductions. Here, Dr Rob Bellamy from the Department of Geography explains his recent research into how BECCS might be incentivised responsibly, by accounting for public and stakeholder preferences
- Nearly 80% of the British public know nothing or only a little about BECCS. However, once it is explained to them, 72.8% of participants were supportive.
- Public acceptability of BECCS will be determined as much by the ways in which it is incentivised and governed as by its technical characteristics. Policymakers need to be mindful of the unintended consequences their choices can have.
- Stakeholder policy preferences reflect geographically varying societal values and interests. Policymakers need to account for the diversity of these perspectives that will ultimately determine the success—or failure—of their policy designs.
What is BECCS and why are public and stakeholder attitudes important?
Bioenergy with carbon capture and storage (BECCS) is an approach to carbon dioxide removal (CDR) which involves generating energy through burning biomass (such as wood and agricultural products, solid waste, landfill gas and biogas or ethanol and biodiesel) coupled with the capture and storage of the resulting CO2, in geological or other long-term reservoirs, such as depleted oil and gas reservoirs.
Despite growing interest in BECCS, it will not come forward without strong institutional support and significant new incentives for research, development, demonstration and deployment. One particularly understudied question concerns how it might be incentivised responsibly, by accounting for public and stakeholder preferences for policy development. This is an urgent question to address, given that BECCS forms a significant part of plans to reach net zero, and that it is unlikely to be rolled out widely if decision makers do not take advantage of the social intelligence that can be gleaned from diverse citizens, industries, non-governmental organisations, scientists, and other governmental policymakers and officials.
Our research
Our two studies set out to explore diverse public and stakeholder preferences with respect to the development of policy for BECCS.
In the first study, we used a combination of quantitative and qualitative methods to compare public perceptions of BECCS in three different policy scenarios. The motivation behind this approach was to understand how alternative policy scenarios might affect perceptions of that technology. We found that the type of policy instrument used to incentivise BECCS significantly affected perceptions of the technology itself. In particular, there was a great deal of opposition towards a price guarantee scheme – where government would guarantee a higher price for producers selling energy derived from BECCS. This stemmed from participants’ knowledge of the high costs being imposed on taxpayers by this mechanism, in order to support new nuclear energy provision (notably in relation to Hinkley Point C).
On the other hand, we found a high level of support for another supportive instrument, fixed payments (whereby government would pay a fixed amount to operators of BECCS based on how much CO2 they remove from the atmosphere), which were the single highest ranked instrument in the study, owing to their ability to establish a direct link between public spending and the climate change performance of BECCS operators.
In the second study, we undertook a comparative analysis of stakeholder attitudes to four idealised policy scenarios for BECCS, including representatives of government, business, nongovernmental and academic communities, in the UK and Sweden. The motivation here was to account for diverse and geographically varying societal values and interests.
Our analysis revealed five key insights. First, a business-as-usual scenario is inconsistent with ambitions to develop and deploy BECCS. Second, any policy incentives to stimulate BECCS should not detract from emissions reductions. Third, economic incentives that focus on carbon pricing risk being too low to stimulate investment in BECCS. Fourth, a dilemma exists with respect to the pursuit of either technology specific and technology neutral policies, where a technology specific policy would incentivise BECCS, but disadvantage other approaches to CDR as well as fossil CCS; while on the other hand, a technology neutral policy could incentivise other CDR approaches and fossil CCS, but disadvantage BECCS as a comparatively immature approach. Fifth, each national context raises different geographical and policy preferences and concerns. These include not only clear geographical contrasts – for example between the two countries’ CO2 storage capacities and biomass supplies – but also differing societal contexts that shape prevailing public policy preferences and stakeholder concerns. For example, British stakeholders showed a particularly strong support for a price guarantee scheme, which is interestingly the very same instrument opposed by British publics.
What does this mean for policymakers?
In light of these findings, we have six key recommendations for policymakers:
- Do not rely only on evidence about attitudes towards the technical characteristics of BECCS, but understand that attitudes will be formed based on the coupled socio-technical systems within which technical features are embedded.
- Our study has identified a number of specific policy contexts for BECCS which have demonstrable potential for broad public support or opposition, including fixed payments which were approved of, and price guarantees which were strongly opposed.
- Recognise the need to develop new incentives and make enabling reforms to existing policy instruments.
- Stakeholders are alert to the importance of avoiding trade-offs between emissions reductions and CDR, suggesting that concerns about mitigation deterrence should be addressed in real-world policy contexts and not in relation to abstracted models.
- Employ multi-instrument approaches to incentivisation that do not overly rely on carbon pricing or force a choice between technology-specific and technology-neutral policies.
- Attend to the diversity of stakeholder and wider public perspectives that will ultimately determine the success—or failure—of their policy designs.
Dr Rob Bellamy is Presidential Fellow in Climate and Society in the Department of Geography at The University of Manchester. His research focusses on the social science of climate, nature, and technology.
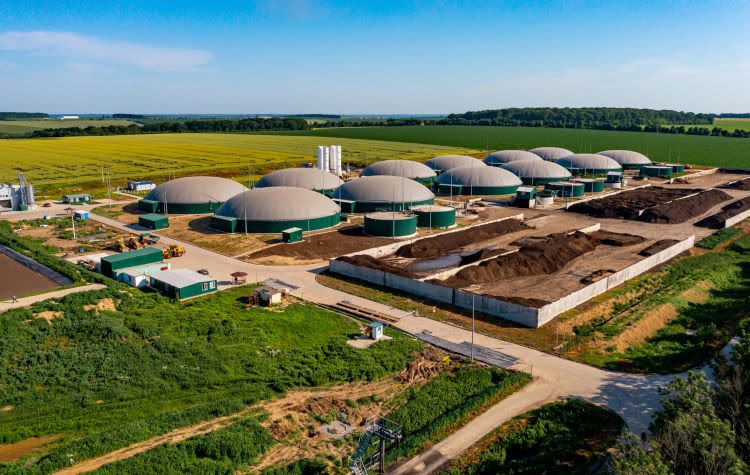
Carbon capture and storage: A solution under our noses
Cathy Hollis, Kevin Taylor and Lin Ma
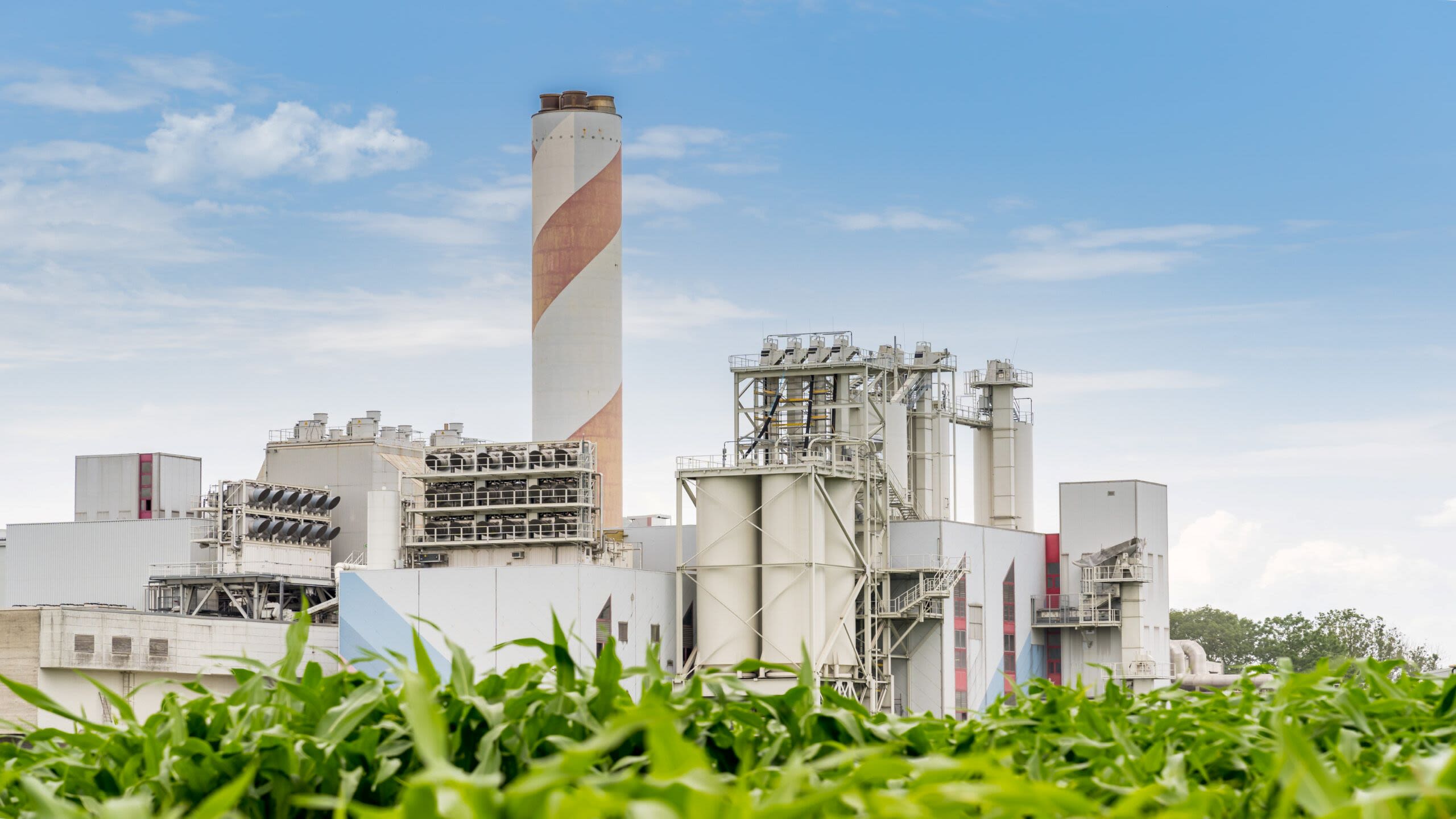
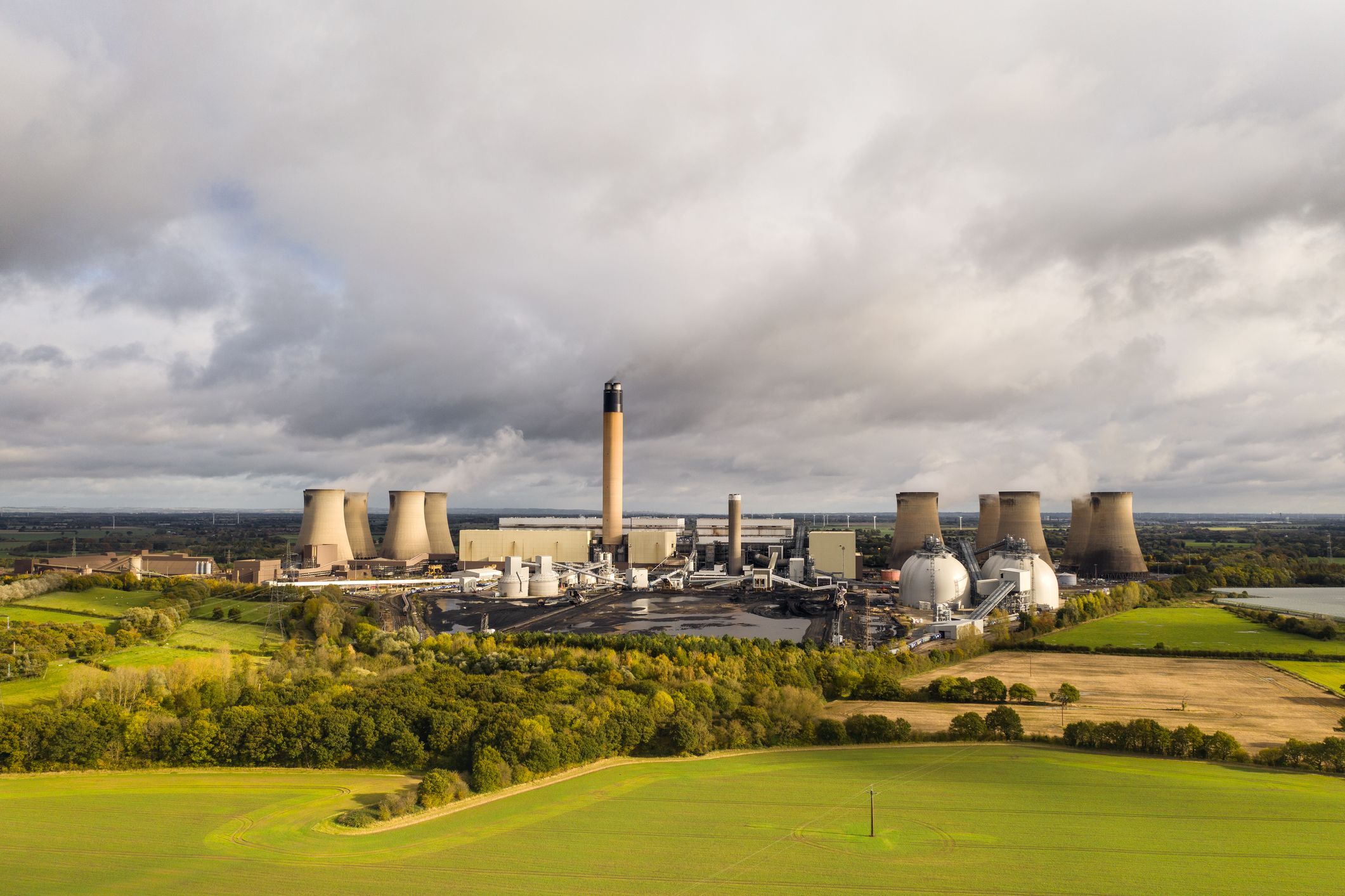
In 2019, the UK became the first major economy to legally commit to achieving net zero CO2 emissions by 2050. With new evidence of rapid, anthropogenic-induced climate change emerging, it is likely that many climate forecasts have underestimated the speed and extent of climate change. This means that drastic action is needed to both slow emissions and remove carbon from the atmosphere. In this blog, Professor Cathy Hollis, Professor Kevin Taylor, and Dr Lin Ma outline the challenges and opportunities for the UK in carbon capture and storage (CCS) technologies, including a role for the oil and gas sector.
- Carbon capture will be vital in limiting global warming to less than 1.5⁰C over the next century.
- There are currently at least 19 CCS facilities either operating or in development in the UK.
- Depleted oil and gas reservoirs, such as those in the North Sea, are ideal for long-term storage of CO2 – but require detailed understanding of potential leakage points.
- Policymakers can support and grow the UK’s CCS industry by ensuring it is integrated into net zero solutions, and making oil and gas exploration data available to researchers.
Carbon Capture, Utilization and Storage (CCUS) is the process of capturing carbon dioxide (CO2) from industrial processes, or potentially directly from the atmosphere, so that it can be recycled for further use or removed to the subsurface. It is one of several negative emission technologies (NETs) that can help achieve net zero. Geological Carbon Storage (GCS) refers to the injection of captured CO2 into the subsurface to store it permanently, and is the most technologically mature and commercially viable technique for CO2 storage. GCS has been actioned for over twenty years with a current storage rate of approximately 3.7–4.2 Mt CO2 a year. The number of projects, and storage capacity, is expected to increase significantly in coming decades with the potential to store up to 25,000 Gt CO2 globally; this is more than sufficient to limit warming to less than 1.5°C through to the year 2100.
How does Geological Carbon Storage work?
CCUS involves three steps: carbon capture, transport from the source to utilisation or storage sites, then consumption in the industrial process, or injection and storage in a secure geological formation. Typically, GCS refers to the final step, which involves direct and permanent storage of CO2 in depleted oil and gas reservoirs, water saturated aquifers, salt caverns, or deep coal seams that have not been mined. There are several key criteria for successful direct storage, the most important being that there is physical space to store CO2, and an overlying impermeable seal to the geological formation to contain the CO2. This seal prevents leakage to the surface. The CO2 is injected directly into the host rock, where it migrates locally via fractures or pore spaces in the rock unit. Understanding the size, connectivity and complexity of this pore or fracture network in the host rock, and the sealing properties of overlying strata, is critical to the success of GCS. High-resolution, and multi-scale imaging at The University of Manchester is allowing this to be conducted on a wide range of geological materials at multiple scales. Furthermore, in some cases, such as limestone formations or volcanic rocks, CO2 can be reacted with the rock and captured as mineral cements. This process is known to occur naturally in geological systems and is now being used on anthropogenic timescales to permanently store CO2.
The most promising locations for permanent CO2 storage are depleted oil and gas reservoirs, and many projects are now underway. These have numerous advantages: there is storage capacity for large volumes of CO2 within pores that previously stored hydrocarbon, the reservoirs have proven seals and traps (having retained millions of cubic metres of hydrocarbon over millions of years), and the well and pipeline infrastructure for CO2 injection is in place. The reservoirs typically occur at depths of more than 1.5km beneath the surface, usually away from urban areas and often offshore. One challenge is to ensure that injected CO2 does not leak through existing boreholes to the surface, and therefore new latex-based ‘smart cements’ are being designed to seal boreholes. At a larger scale, research at The University of Manchester is using historical well and seismic data to map seals and determine potential leakage points in North Sea reservoirs leading to development of a confidence matrix for mapping potential leakage points.
Such detailed geological analysis is critical to the safe and secure storage of CO2, and requires that legacy data from over 50 years of UK oil and gas exploration and production is retained and made accessible to researchers by the government.
What are the challenges of Geological Carbon Storage work so far?
The high cost and low-economic benefit of CCUS, including GCS, has been a major challenge to industry, and it is clear that government support is critical to integrating CCUS into net zero solutions. In 2021, the UK government announced £200 million funding to support the development of at least two carbon capture and storage hub and cluster projects across the UK by the end of the decade. This commitment is essential to the success of blue hydrogen production, which promises a key fuel for future energy use in the UK, but which generates CO2 during production. Potential sites for hydrogen production include HyNet North West in NW England and North Wales, which are ideally located for storage of industrially-produced CO2 offshore in depleted gas reservoirs. The scope of work and roadmap to achieve the Green Industrial Revolution are now being rolled out and details are still to be made public.
What is clear is that future solutions will require close collaboration between subsurface engineers and geoscientists, production engineering and the public. Despite the clear benefits to greenhouse gas reduction, there is still uncertainty in the public arena as to the safety and effectiveness of GCS. In particular, although the need for a reduction in atmospheric CO2 is well understood, some people are concerned about induced earthquakes, leakage of CO2 and potential hazards to marine life, whilst others fear that it might delay decarbonisation. Some of these concerns can be addressed through advanced atmospheric monitoring and continued public engagement.
It is critical that the geoscience community and the legacy of subsurface research, such as that conducted at The University of Manchester, remains part of the dialogue with government agencies, industry and the public.
Cathy Hollis is Chair of Carbonate Geoscience at The University of Manchester. Her work focuses on characterisation of pore networks in geological formations, and their control on fluid flow and reactions, particularly in the context of hydrocarbon production, geothermal energy, carbon sequestration and gas storage.
Professor Kevin Taylor is a lecturer in Geoscience. He has research links and interests in subsurface energy and the utilisation of the sub-surface for the decarbonisation of energy, storage of heat and energy, and in long term safe storage and disposal of CO2 and nuclear waste. His interests in the hydrogen economy focus around understanding the challenges that are presented by storage of hydrogen (and other gases) in porous rocks in the sub-surface (efficiency, mobility risks, and leakage risks) and he utilises multi-scale 3D imaging and rock characterisation to address these.
Lin Ma is a NERC Research fellow and Presidential Fellow, whose work uses multi-scale imaging for characterising rocks in geoenergy reservoirs and subsurface energy storage. She is currently developing novel approaches to characterise pore networks and rock properties, and how they react under subsurface conditions.
The hydrogen economy: why is the sub-surface essential?
Kevin Taylor
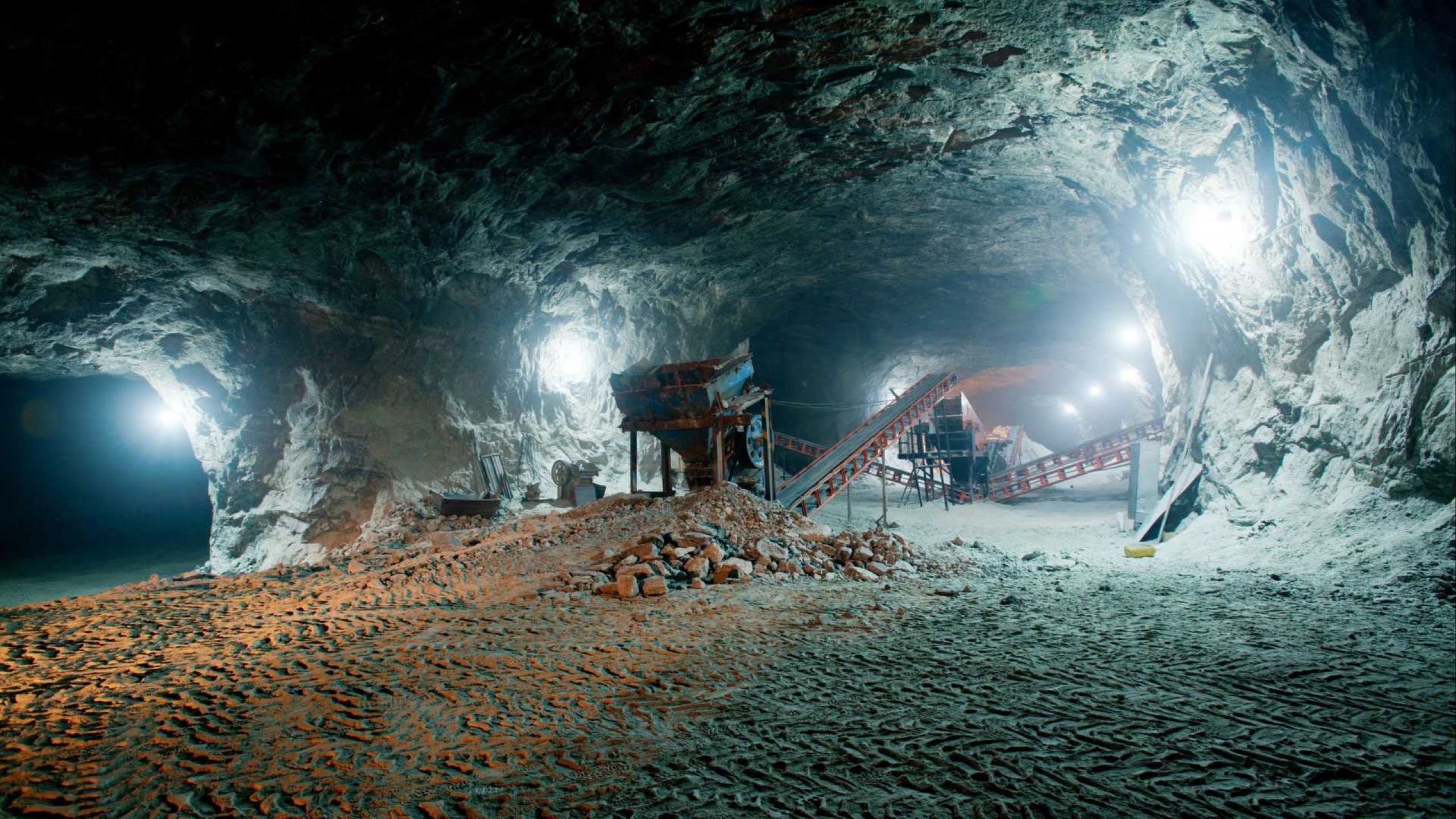
The UK Government has made a commitment to deliver a hydrogen economy as a means to decarbonise heating and heavy transport. This was most recently highlighted in the Government’s “Ten-point plan for a green industrial revolution” and the recent Energy White Paper “Powering our net zero future”. In this blog, Professor Kevin Taylor, from the Manchester Environmental Research Institute, lays out the role of the sub-surface in both hydrogen storage and carbon capture – both crucial strands of the UK’s net zero ambitions.
- Most hydrogen production currently generates CO2 as a by-product, which needs to be stored via carbon capture and storage.
- Once produced, hydrogen gas will also need the be stored temporarily or seasonally to address demand fluctuations.
- The sub-surface can meet both of these roles using existing natural formations, such as deeply buried sandstones offshore or salt caverns in Cheshire.
- Policymakers must implement a nation-wide pilot programme to identify more suitable sites, and ensure the UK has the skills and infrastructure needed to make sub-surface storage a reality.
The government’s commitment to a hydrogen economy has led to promises of a number of hydrogen networks in the UK, a potential “hydrogen town” by the end of the decade, and of greater investment across the energy sector to meet these targets. However, this ambition is not without its challenges and risks. As a geoscientist, it is clear to me that two aspects where we will need significant and rapid investment, leading to development and implementation of technology, are at the end of the hydrogen economy chain – underground (or geological) capture and storage (CCS) – and the very likely need to temporarily store hydrogen in the sub-surface to buffer intermittent energy demands. While on the face of it these appear to be different challenges, they share a great deal in common.
Currently, most of the global production of hydrogen is manufactured from coal (so-called brown hydrogen). This process not only fuels the coal economy, but also produces significant amounts of CO2 as a by-product. An alternative source is through the manufacture of hydrogen from natural methane gas, via a process called steam methane reformation; so-called grey hydrogen. While this is “cleaner” than brown hydrogen, it still produces CO2 as a by-product. Much store has been placed, at least in the next two decades, in burying this CO2 in the ground (carbon capture and storage – CCS), thereby locking the CO2 away long-term (blue hydrogen). The ultimate goal, of course, is for green hydrogen to be produced utilising renewable energy sources and the electrolysis of water, which releases no CO2. However, the economics of green hydrogen production, and the relative abundance and cheaper availability of producing it from methane, has led many to conclude that CCS will need to be a key part of the hydrogen economy supply chain for the foreseeable future.
Why use the sub-surface for storage and disposal?
To many the sub-surface has traditionally been associated with the extraction of fossil fuels, and so it is not immediately seen by the public to be part of the solution to meeting net zero. However, the sub-surface can and should play a key role in safely disposing of CO2. We know that two potential parts of the sub-surface could hold and retain CO2; either spent oil and gas reservoirs, or deep saline aquifers. Currently, the UK has no active CCS sites operational – plans to invest in CCS pilot sites were abandoned in 2015 due to insufficient fiscal support from the previous government. In contrast, there have been a number of successful CCS projects in the Norwegian sector of the North Sea (eg the Sleipner CCS site) and new projects have recently been announced (such as the Northern Lights Project). According to the IEA, globally, there are still only around 20 projects in commercial use, but in the last three years, plans for more than 30 commercial carbon capture facilities have come forward, representing a potential investment of about $27bn (£20.7bn). The UK, for example, has announced two new projects in Teesside and Humberside involving consortia of energy and engineering companies.
The ability of the sub-surface to trap gas has also led to proposals for the temporary sub-surface storage of hydrogen, in order to off-set fluctuating/seasonal demand. These could be simple engineered tanks buried in the ground, but why go to the expense of this when rocks in the sub-surface have the capability to have gases pumped in and pumped out, as evidenced by a number of existing gas storage projects in the UK and wider. A recent assessment has suggested that gas fields under the North Sea could provide this storage. Here, the northwest of England could also help to offer sub-surface storage solutions with the presence of highly suitable mined-out salt caverns in Cheshire.
Integration in the northwest of England
The northwest of England has a hydrogen economy project that is being developed with a clear ambition to deliver both hydrogen and a path to net zero by burying CO2 produced via hydrogen production, and utilising temporary hydrogen storage in the sub-surface. Sites that may be suitable for the former have been identified in the sub-surface under the Irish Sea, where there are existing hydrocarbon gas sub-surface storage systems, in addition to the already mentioned potential for hydrogen storage in Cheshire salt caverns.
It is clear that the sub-surface is a key player in the hydrogen economy, and geoscience and engineering technology and skills, which already exist in many parts of the energy sector, will be critical to delivering the hydrogen economy promised by the UK Government. Policymakers should work with established experts in this field, such as oil and gas geologists and engineers, to reduce the skills gap needed to properly utilise the potential of the sub-surface.
The northwest of England is in an excellent position to take advantage of key areas beneath our feet. Investment and research towards better understanding this sub-surface domain – and how it will behave when gases are pumped into, stored and retrieved from it – will be needed here in the form of research pilot sites where independent and verifiable trials can take place are needed. Again, the northwest of England has a head-start in this. The British Geological Survey, together with the UKRI, have planned a UK Geoenergy Observatory in Cheshire, (UKGEOS) which will use data from lab studies on rock core from the sub-surface, combined with 3D mapping and in-situ real time monitoring data to reduce uncertainties. But given the variable geology of the sub-surface, both regionally and nationally, a policy of wider study should be a priority. We are on the road to hydrogen, but there is much to be done if we are to realise this exciting potential.
Professor Kevin Taylor is a lecturer in Geoscience. He has research links and interests in subsurface energy and the utilisation of the sub-surface for the decarbonisation of energy, storage of heat and energy, and in long term safe storage and disposal of CO2 and nuclear waste. His interests in the hydrogen economy focus around understanding the challenges that are presented by storage of hydrogen (and other gases) in porous rocks in the sub-surface (efficiency, mobility risks, and leakage risks) and he utilises multi-scale 3D imaging and rock characterisation to address these.
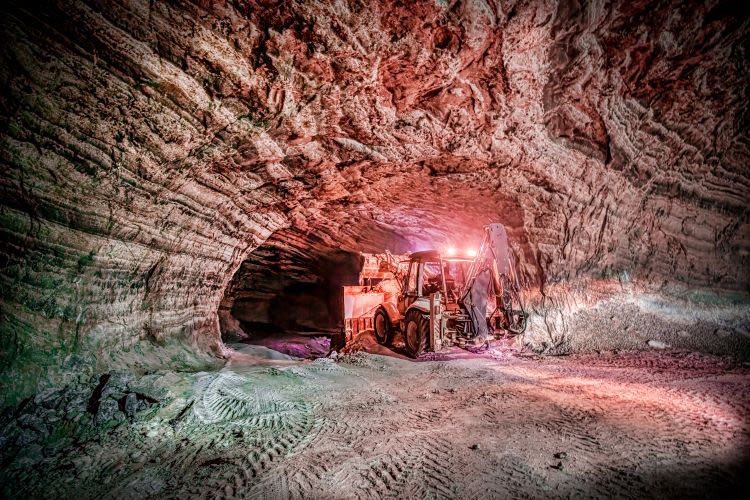
Bioenergy with Carbon Capture and Storage – Real-worlding emission estimates
Muir Freer, Clair Gough and Andrew Welfle
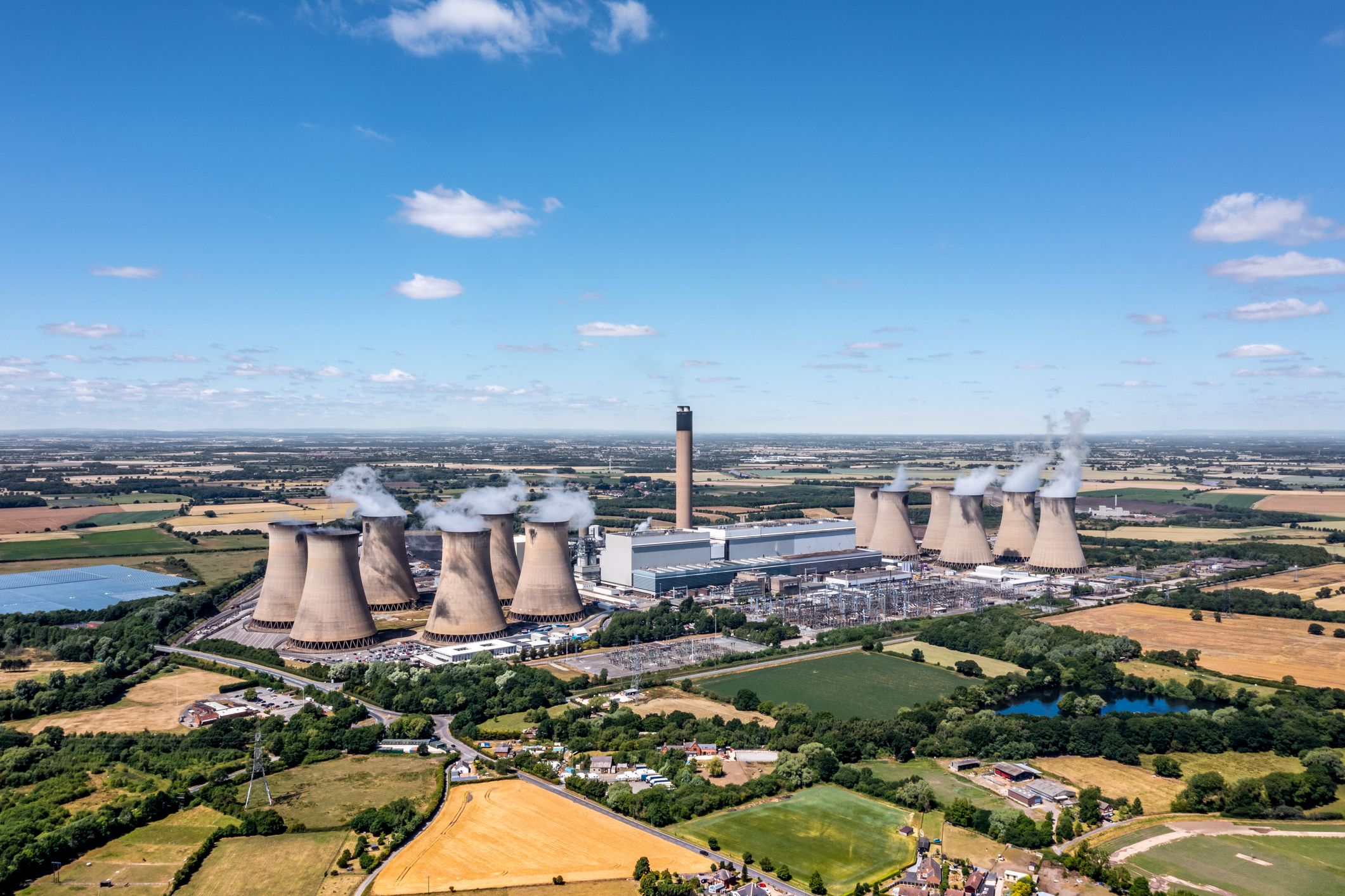
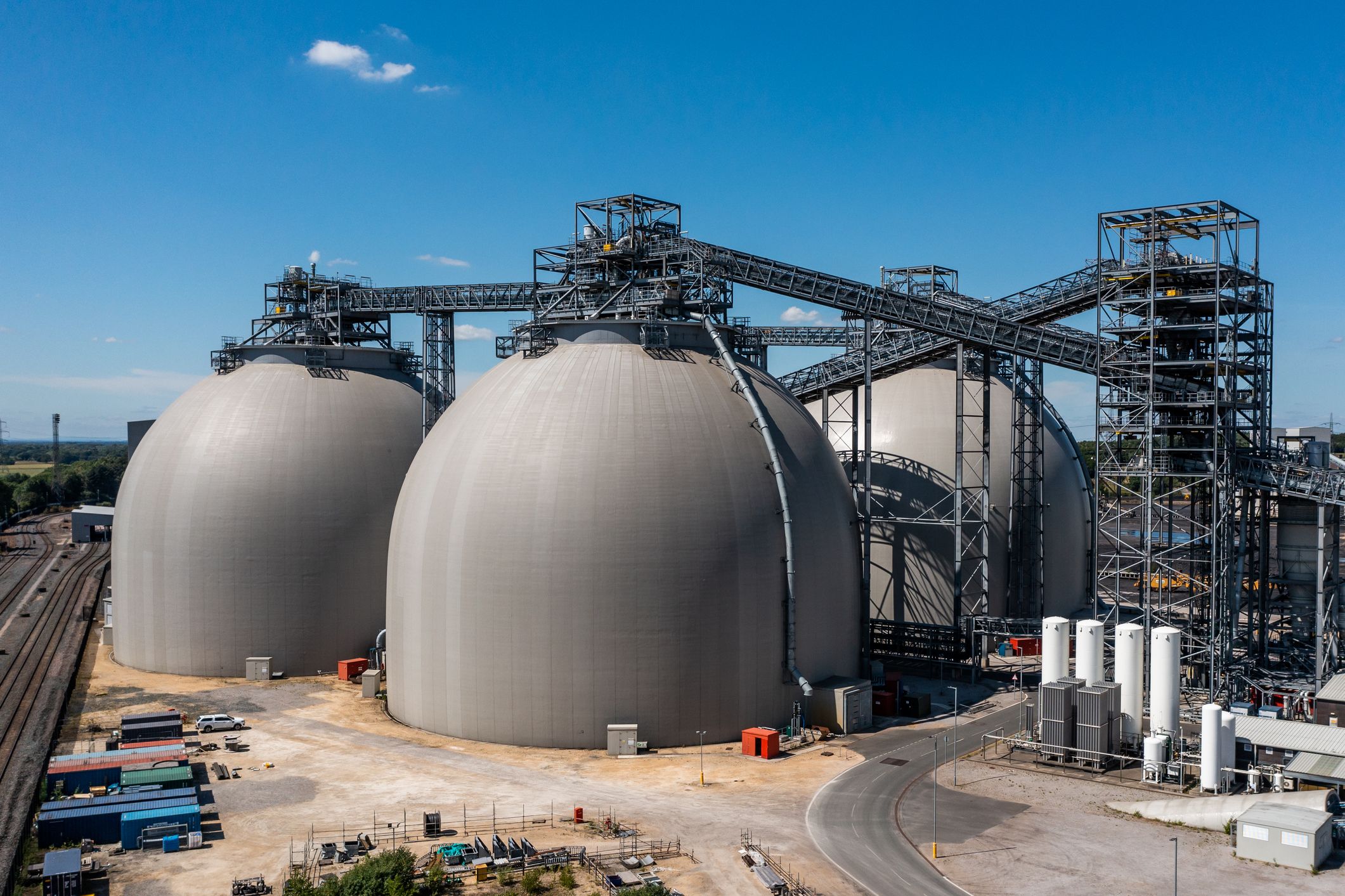
BECCS is an important Carbon Dioxide Removal (CDR) technology, which consists of the combustion of biomass ranging from agricultural residues to industrial wastes and energy crops while producing biofuels, power and hydrogen, and capturing the emissions and securely storing them below ground. The technology is considered key to achieve net zero as it has been projected to remove up to 96.5 MtCO2 per year within the UK by 2050 depending on the scenario.
Some areas of the UK are better suited for certain configurations of BECCS supply chains depending on their access to biomass, geological storage, their energy users and low-carbon transportation infrastructure. For example, agricultural residue derived BECCS-Power supply chains are well suited for the Humber cluster, while BECCS-Waste-to-Energy supply chains are well suited for the North-West cluster.
Moreover, some areas are better suited to support large-scale BECCS facilities than others hence the importance of determining the optimal BECCS facility scale. For example, the average optimal facility scale of a wheat straw derived BECCS-Power supply chain ranges from 0.87 to 1.38 MtCO2 per year in the Humber region and 0.59 to 1.09 MtCO2 per year in North Wales.
We created the Carbon Navigation System (CNS) model and Biomass Repository to aid decision making for policymakers and industry to better organise and plan the implementation of BECCS for the UK to meet net zero more sustainably.
How does the model work?
Say you wanted to go to the cinema, Google Maps would tell you to walk for 5 minutes, take a bus for 20 minutes and then walk for a further 5 minutes to reach your destination. The CNS model works in a similar way, but where Google Maps optimises transportation times for people, it optimises CO2 transportation emission for BECCS facilities.
For any industrial cluster or dispersed location in the UK, the model can simulate the transport characteristics of any BECCS supply chain while automatically switching between pipeline and non-pipeline transportation methods to minimise emissions. For example, the same wheat straw derived BECCS-Power supply chain calibrated to capture 1 MtCO2 per year has been simulated in two different locations in the diagram below, where the supply chain transportation emission are 7.6 times greater in the dispersed cluster siting location compared to the pipeline cluster location.
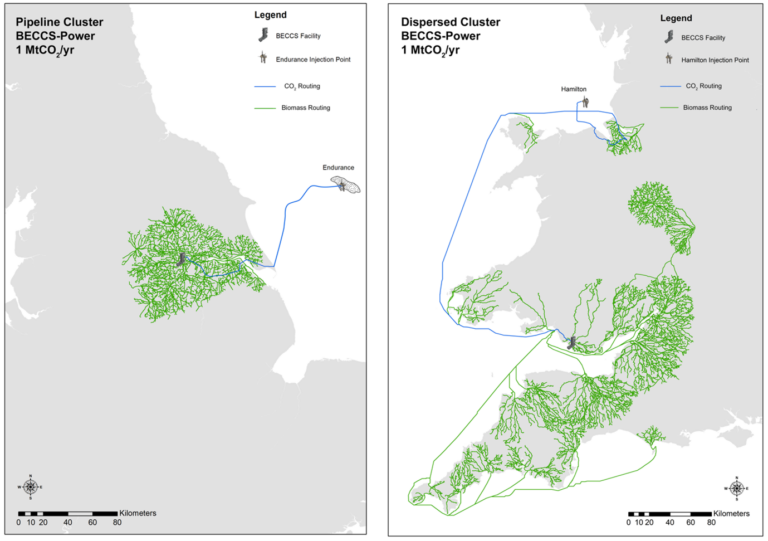
Simulation of a wheat straw derived BECCS-Power supply chain calibrated to capture 1 MtCO2 per year simulated within a pipeline cluster location and a dispersed cluster location.
Simulation of a wheat straw derived BECCS-Power supply chain calibrated to capture 1 MtCO2 per year simulated within a pipeline cluster location and a dispersed cluster location.
The Biomass Repository was developed alongside the CNS model, as a high resolution database which details where the biomass types are and what the potential resource is at each site. When this database is integrated into the CNS model, the model can search for a specific quantity of biomass at a chosen BECCS facility location, and will estimate transportation emissions for the selected site.
From that same location, the model can calculate how much CO2 can be captured from the biomass and will calculate the route for the facility to be linked up with one of the industrial CCS clusters to securely and permanently store CO2 offshore. Any energy or fuel generated by the facility can also be transported to its users or energy storage method, such as integration with the national grid.
Moreover, the supply chains can be modelled under certain biomass security scenarios – an increase in competition from other sectors for biomass supply, the closure of domestic biomass suppliers, biomass supply shocks from heatwaves and droughts and increased competition from other domestic or international BECCS supply chains – to determine the impact on their carbon performance. For example, a 50% decrease in biomass yield would result in an average 5-10% increase in transportation emissions for BECCS supply chains in the UK.
The model can be repurposed to model any CCS or other supply chain (bio-chemical, glass, cement and energy sectors, for example). It is especially suited for connecting different emitters across the UK to geological storage sites and was designed to plug into any life cycle analysis to reduce transport emissions and better base their analysis in reality.
Aiding decision making
The CNS model can reduce the uncertainty in the multitude of factors which can impact the deployment potential of BECCS for the UK to meet net zero, including optimal sitting locations, optimal facility scales for specific areas in the UK, the impact of biomass security and the impact from the decarbonisation of the transport sector.
The analysis will allow policymakers, academia and industry to identify and quantify the impact on the carbon performance of BECCS supply chains for the major sources of uncertainty for BECCS deployment and help improve potential deployment pathways and roadmaps to achieve net zero sustainably.
A greater understanding of the prioritisation of transportation decarbonisation pathways for BECCS supply chains can be gained from the CNS model scenarios. For example, transitioning to a high degree decarbonised HGV fuel like green hydrogen (8gCO2/t-km) would result in a 73-74% decrease in BECCS supply chain transportation emissions for the UK, indicating that the decarbonisation of HGVs within BECCS supply chains have the greatest potential carbon savings as the integration of high degree decarbonised fuels into shipping and rail reduce transportation emissions by 35-36% and 29-33%, respectively.
Policy recommendations
- The CNS model and Biomass Repository aids decision making for policymakers and industry by simulating BECCS supply chains over a wide range of locations to determine their carbon performances across the UK.
- BECCS supply chains must be carefully sited and integrated into their wider infrastructural, geographical, economic and social landscapes to ensure their low-carbon deployment.
- To account for these multiple factors, we have developed a model which determines the location enabling the least carbon emissions throughout the entirety of the supply chain while determining the optimal facility scale and accounting for different biomass security scenarios.
- Importantly, results indicate that the decarbonisation of HGVs within BECCS supply chains have the greatest potential carbon savings.
Dr Muir Freer is a Research Associate based at the Tyndall Centre for Climate Change Research. His research focuses on modelling CCUS and BECCS supply chains for the UK at high spatial resolution and connecting dispersed emitters to offshore geological storage.
Dr Clair Gough is a Senior Research Fellow with the Tyndall Centre for Climate Change Research. Her research has integrated technical and social science analyses in the context of energy and climate change, focusing on the assessment of carbon dioxide capture and storage (CCS), including the use of biomass energy with CCS (BECCS).
Dr Andrew Welfle is a Senior Research Fellow at The University of Manchester, based in Tyndall Centre for Climate Change Research. Andrew is also part of the UK Supergen Bioenergy Hub research programme.
Analysis and ideas on carbon capture and storage policy, curated by Policy@Manchester
With special thanks to our contributors:
Dr Sarah Mander is a Reader and theme coordinator for Behaviour and Governance at the Tyndall Centre at The University of Manchester. Her research is focused on climate change mitigation and she has researched renewable energy, long term energy scenarios, sustainable energy in the urban environment, recycling and climate change governance.
Dr Clair Gough is a Senior Research Fellow with the Tyndall Centre for Climate Change Research. Her research has integrated technical and social science analyses in the context of energy and climate change, focusing on the assessment of carbon dioxide capture and storage (CCS), including the use of biomass energy with CCS (BECCS).
Dr Rob Bellamy is Presidential Fellow in Climate and Society in the Department of Geography at The University of Manchester. His research focusses on the social science of climate, nature, and technology.
Cathy Hollis is Chair of Carbonate Geoscience at The University of Manchester. Her work focuses on characterisation of pore networks in geological formations, and their control on fluid flow and reactions, particularly in the context of hydrocarbon production, geothermal energy, carbon sequestration and gas storage.
Professor Kevin Taylor is a lecturer in Geoscience. He has research links and interests in subsurface energy and the utilisation of the sub-surface for the decarbonisation of energy, storage of heat and energy, and in long term safe storage and disposal of CO2 and nuclear waste. His interests in the hydrogen economy focus around understanding the challenges that are presented by storage of hydrogen (and other gases) in porous rocks in the sub-surface (efficiency, mobility risks, and leakage risks) and he utilises multi-scale 3D imaging and rock characterisation to address these.
Lin Ma is a NERC Research fellow and Presidential Fellow, whose work uses multi-scale imaging for characterising rocks in geoenergy reservoirs and subsurface energy storage. She is currently developing novel approaches to characterise pore networks and rock properties, and how they react under subsurface conditions.
Dr Muir Freer is a Research Associate based at the Tyndall Centre for Climate Change Research. His research focuses on modelling CCUS and BECCS supply chains for the UK at high spatial resolution and connecting dispersed emitters to offshore geological storage.
Dr Andrew Welfle is a Senior Research Fellow at The University of Manchester, based in Tyndall Centre for Climate Change Research. Andrew is also part of the UK Supergen Bioenergy Hub research programme.
All opinions and recommendations made by article authors are made on the basis of their research evidence and experience in their fields. Evidence and further discussion can be obtained by correspondence with the authors; please contact policy@manchester.ac.uk in the first instance.
Read more and join the debate at: blog.policy.manchester.ac.uk
www.policy.manchester.ac.uk
October 2022
The University of Manchester
Oxford Road, Manchester
M13 9PL
United Kingdom
The opinions and views expressed in this publication are those of the respective authors and do not necessarily reflect the views of The University of Manchester.
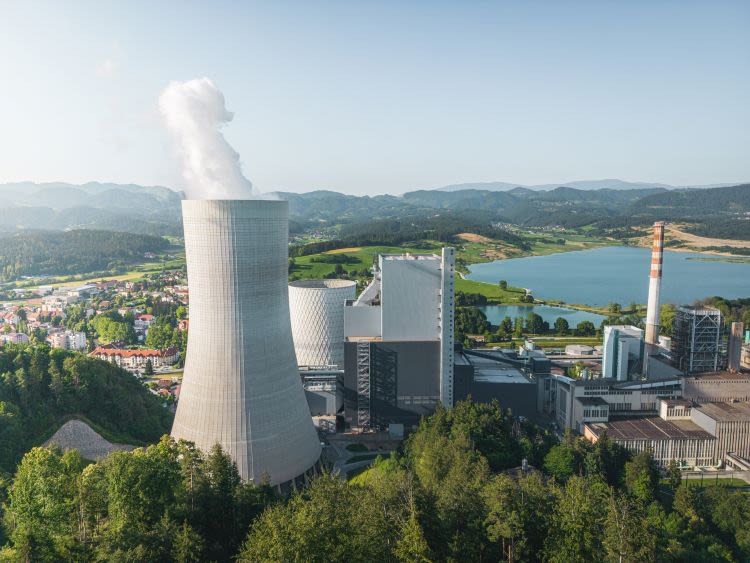